Spray Drying: Solving solubility issues with amorphous solid dispersions
Posted: 3 September 2015 |
Amorphous solid dispersions (ASDs) are increasingly being used as a means of improving bioavailability of poorly water-soluble compounds in research and development, and spray drying technology has been recognised as one of the useful methods to generate ASDs.
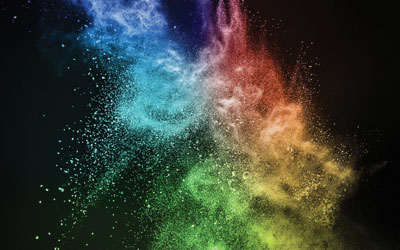
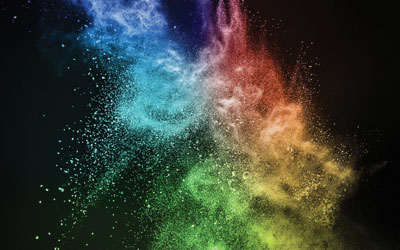
Although the application of spray drying for the production of amorphous solid dispersions in the drug discovery stage is still limited, amorphous solid dispersions prepared with a small-scale spray drying process can be an effective approach to deliver high doses of poorly water-soluble compounds and to enhance their plasma exposure in in vivo studies for early drug discovery efforts. This article reviews the application of this technology for the production of amorphous solid dispersions in a single process, and the use of small-scale spray dryers to produce amorphous solid dispersions as early preclinical formulations using only milligram quantities of drug substances.
Early preclinical formulations
It is estimated that 40 to 60% of drug compounds in today’s drug discovery phases exhibit poor aqueous solubility, and these compounds frequently have certain delivery limitations. Early preclinical formulations are developed for in vivo pharmacology, safety pharmacology and toxicology studies in drug discovery. The main purpose of these formulations is to obtain sufficient plasma exposure in in vivo studies for optimising, selecting and advancing compounds to the clinic. However, inadequate plasma exposure does not sufficiently provide safety margins surrounding the predicted efficacious dose in animal models.
Oral gavage is the most common route in animal dosing, and in general, solution formulations are preferred due to their simplicity, dose accuracy and dose flexibility. For poorly water-soluble compounds, enabling solution formulations with solubility enhancement using a co-solvent, cyclodextrin, surfactant, lipid-based product, or the combination formulations, are employed to yield improved plasma exposures. However, these enabling solution formulations often have limitations, such as poorly soluble compounds failing to reach target drug concentrations. Drug precipitation and vehicle-related side effects can also occur. These issues limit the amount of the poorly soluble compounds that can be administered to animals as a solution.
Conventional drug suspensions are capable of providing high drug concentrations, but suspensions of poorly water-soluble compounds often exhibit dissolution rate-limited drug absorption. In addition, conventional suspensions of different batches produced in the drug discovery stage often have batch-to-batch inconsistency, which can cause significant variability of dissolution and bioavailability. Crystalline nanosuspensions are frequently introduced as enabling suspensions to solve the dissolution rate issues. Meanwhile, nanosuspensions have shown significant improvement in enhancing drug dissolution and bioavailability. However, the batch-to-batch inconsistency of drug substances supplied in the drug discovery stage and solubility-limited absorption for high doses of poorly soluble compounds are the limitations in the application of crystalline nanosuspensions in drug discovery. That is where ASDs come to the fore – they offer an alternative and effective way to deliver high doses and enhance in vivo exposure of poorly soluble compounds.
Benefits of amorphous solid dispersions
A solid dispersion (SD) is defined as the dispersion of a drug compound in a carrier matrix (often polymers) at solid state. In the case of an ASD, a crystalline form of the compound is converted to an amorphous form, which is dispersed in, and stabilised by, a carrier. By eliminating the inherent solubility limitations associated with the crystalline form, the ASD offers the solubility benefits of a solution formulation without the need for non-aqueous vehicles, which can limit dosing volumes. Additionally, the dissolved carrier excipient(s) in solution delays precipitation and maintains supersaturation of the compound in the gastrointestinal tract. When supersaturation is sustained, the absorption of the compound can be maximised to be greater than that of a saturated solution.
While ASDs have been increasingly used to enhance bioavailability of poorly soluble compounds at a later stage of the drug development process, this approach has had limited utility at the stage of drug discovery, i.e., it has tended to be used only as a last resort. This is primarily because preparing reliable ASDs at the milligram scale is technically challenging, and therefore would require considerable development time and amount of drug substance. Among the several different manufacturing methods explored to produce ASDs, hot melt extrusion (HME) and solvent evaporation are the two lead approaches to enable poorly soluble compounds. HME is not suitable in the drug discovery stage due to its requiring a relatively large quantity of drug substance. For the small-scale preparation of an ASD, rotary solvent evaporation is commonly used. However, the stressed conditions (i.e., the gradual solvent evaporation process at an elevated temperature with a relatively long evaporation time) employed by this method often lead to the chemical instability of the drug compound and also drug–carrier phase separation of the ASD. In addition, the dried solid material will typically be in the form of a film, and thus post-processing techniques (isolating, milling and sieving) are necessary to obtain the desired properties of ASD powders. From a scalability and process perspective, rotary evaporation is not an ideal method in the drug discovery stage.
Application of the spray drying technology
Spray drying is a continuous and scalable solvent evaporation method. It converts solutions to powders in a single process, and it has been used for the preparation of ASDs of poorly soluble compounds. The spray drying process involves: 1) dissolving the drug and the carrier excipient(s) in a common solvent to prepare a feed solution; 2) pumping the feed solution into a spray nozzle; 3) atomising the solution stream into fine droplets via an appropriate device; 4) drying the fine droplets in a drying chamber; and 5) separating and collecting the dried powders via a suitable collector.
Compared to rotary evaporation and HME, the spray drying process gives us more diverse options for ASD carrier excipient(s). For instance, the cellulose-based polymers can be used as ASD carriers by the spray drying process, but it is difficult to use them with the rotary evaporation or HME processes. The solvent system used for the spray drying process should be volatile with good solubilising powder for both the drug and the carrier. The most common solvents are methanol, acetone, or a combination of the two. The solid load and solubility in the solvent system determines the viscosity of the solution, which can have an adverse effect on the atomisation process. Therefore, the total solids load in the feed solution is generally dictated by the solubility of the drug and carrier as well as the viscosity of the solution.
The spray dried ASD powders are yielded from rapid solvent evaporation from droplet surfaces by the atomisation and drying processes. Because the solvent evaporation process is extremely fast (in the order of seconds), spray drying is particularly advantageous for preparing ASDs of compounds with poor thermal stability. Additionally, forming a single-phase mixture of drug-carrier through rapid solidification is particularly important to prevent drug–carrier phase separation. The phase separation can lead to physical instability (e.g., recrystallisation) of an ASD and consequently, poor dissolution and poor bioavailability.
Particle size and properties of ASD powders can be easily optimised through process parameter optimisation of spray drying and/or the type of spray nozzle. The typical properties of spray-dried powders with high surface area and low bulk density are useful to produce a homogenous suspension by constituting ASD powders in aqueous vehicles. Furthermore, the ASD powders made by the process have small particle sizes, so the drug can be easily and accurately dosed via a gavage needle of reasonable gauge by standard animal dosing practices. Accurate dosing in animals is prerequisite to establish dose-response relationship in in vivo studies.
For a more detailed discussion regarding the principles of spray drying and ASDs, the inquiring reader is referred to the ‘Spray Drying Handbook’1 and ‘Amorphous Solid Dispersions: Theory and Practice’ books.2
Small-scale spray dryers applicable to drug discovery
While the spray drying technique has been widely used to prepare ASDs in development, this technology has had limited utility in drug discovery, where bulk drug supply is extremely limited (in milligram quantity). Most of available lab-scale spray dryers have poor yield and require gram (vs. milligram) quantity of material. Recently, two small-scale spray dryers, Büchi Nano Spray Dryer B-90 (B-90, Figure 1; page 00)3 and ProCepT spray dryer/chiller 4M8-TriX (4M8-TriX, Figure 2)4, have become commercially available. As they can generate particles in milligram sample quantities at high yields, they are suited for the use in drug discovery phases. However, there are only a few literature examples describing their use for the preparation of ASDs5,6. The two spray dryers are compared in Table 1 (page 00).
As with other spray dryers, the two small spray dryers use three main steps: atomisation, drying and collection. Although pneumatic two-fluid nozzles are the most common atomisers used in the lab-scale spray dryers due to their simplicity and flexibility, the ultrasonic nozzles, which are also found in the 4M8-TriX unit, offer the advantage of spraying very small amounts of solution with high yields. Unlike other spray dryers, the B-90 unit has a unique piezoelectric driven spray head, which consists of 4, 5.5 or 7μm spray mesh. Millions of precisely sized droplets are ejected from the holes by vibration of the spray mesh (Figure 3; page 00). The small-scale units have small dimensions of the drying chambers, which limit the residence time distribution of atomised droplets. Therefore, the atomised droplets must be small so that they can be easily dried in the drying process prior to exiting the chamber or impacting the chamber walls. The laminar drying gas flow pattern is introduced to the B-90 and 4M8-TriX units to reduce the collision of droplets/particles with the chamber walls and to increase the duration for drying of droplets/particles for obtaining high yields. Another unique feature of these systems is that the drying chamber is constructed of glass for visualisation of the drying process.
Cyclones are the most common collection systems in pharmaceutical spray drying. The 4M8-TriX unit is also equipped with a cyclone, which collects dried particles with particle sizes ranging from 1 to 150µm. The B-90 unit also has an electrostatic particle collector for collecting charged fine particles, which are negatively charged after atomisation (Figure 4; page 00).7 These charged fine particles are discharged after they are deposited onto the inner wall of the cylindrical particle collecting electrode. The B-90 is the only unit capable of producing and collecting particles ranging from 300nm to 5µm at high yields of up to 90% even at small sample quantities in the milligram or milliliter range.
In summary, ASDs of poorly water-soluble compounds can enhance plasma drug exposure and deliver high doses in in vivo animal studies. Moreover, the adverse effect caused by the carriers of ASDs is much less than that of other enabling formulations. The simple spray-drying process is ideal for the production of ASDs with rapid solidification to avoid drug-carrier separation. The recently available small-scale spray dryers offer the opportunity to apply the spray drying technology for the production of ASDs in drug discovery with milligram drug quantities at high yields.
References
- Spray Drying Handbook, authored by Masters, K., Longman, ISBN-13: 978-0582062665 (1991)
- Amorphous Solid Dispersions: Theory and Practice, edited by Shah, N. etc., Springer, DOI: 10.1007/978-1-4939-1598-9 (2014)
- Nano Spray Dryer B-90. http://www.buchi.com/us-en/products/spray-drying-and-encapsulation/nano-spray-dryer-b-90.
- ProCept Spray Dryer/Chiller. http://www.procept.be/spray-dryer-chiller.
- Gu, B, Linehan B, Tseng, YC. Optimization of the Büchi B-90 spray drying process using central composite design for preparation of solid dispersions. International Journal of Pharmaceutics. 2015; 491 (1-2), 208-217, doi:10.1016/j.ijpharm.2015.06.006
- Ormes, JD, Zhang, D, Chen, AM, Hou, S. Krueger, D, Nelson T, Templeton, A. Design of experiments utilization to map the processing capabilities of a micro-spray dryer: particle design and throughput optimization in support of drug discovery. Pharmaceutical Development and Technology. 2013; 18 (1), 121-129, doi:10.3109/10837450.2011.646424
- Lee SH, Heng, D, Ng WK, Chan H-K, Tan RBH. Nano spray drying: a novel method for preparing protein nanoparticles for protein therapy. International Journal of Pharmaceutics. 2011; 403 (1-2), 192-200, doi:10.1016/j.ijpharm.2010.10.012
Biography
|
Dr. Yin-Chao Tseng is a Senior Research Fellow at Boehringer Ingelheim Pharmaceuticals, Inc. in the US. He is currently leading the Discovery Pharmaceutics group, and his key responsibilities include early preclinical formulation development and solid form evaluation for drug candidate nomination for development. Over the course of 23 years working in the pharmaceutical industry, he has been involved in all phases of the drug development process from drug discovery to full development at Wyeth, Bayer and Boehringer Ingelheim.