High throughput electrophysiology platforms as enablers
Posted: 25 January 2007 | | No comments yet
Ion channels play key roles in regulating cardiac, neuronal and secretory tissue function. As such, the pharmacological modulation of ion channel activities may provide an effective means to positively impact upon an array of disease states. However, the reliance on low-capacity manually operated electrophysiology screening systems has dictated that ion channels have remained relatively unexploited as drug targets.
Ion channels play key roles in regulating cardiac, neuronal and secretory tissue function. As such, the pharmacological modulation of ion channel activities may provide an effective means to positively impact upon an array of disease states. However, the reliance on low-capacity manually operated electrophysiology screening systems has dictated that ion channels have remained relatively unexploited as drug targets.
Ion channels play key roles in regulating cardiac, neuronal and secretory tissue function. As such, the pharmacological modulation of ion channel activities may provide an effective means to positively impact upon an array of disease states. However, the reliance on low-capacity manually operated electrophysiology screening systems has dictated that ion channels have remained relatively unexploited as drug targets.
More recently, however, significant advances in electrophysiological screening technologies have resulted in the launch of automated, high-capacity and high-throughput electrophysiology systems that now allow larger compound libraries to be screened relatively rapidly, providing key structure-activity relationship data to support drug discovery programs. As these new platforms are increasingly integrated into drug discovery environments they will undoubtedly help increase the number of focused ion channel drug libraries available and realise the full potential of ion channels as drug targets.
Historical perspective
Ion channels regulate the flow of ions down their electrochemical gradient across a normally ion-impermeable cell membrane. The selective movement of K+, Na+, Ca2+ or Cl- ions, through voltage, ligand, or mechanical activation of these channels, regulates a number of key physiological processes. As such, ion channels are of particular importance in the setting of drug discovery in terms of (i) providing new therapeutic targets and (ii) safety or selectivity hurdles. Consequently, understanding the (patho) physiological role of ion channel families and subtypes, validating them as drug targets and implementing technologies to allow rationale drug discovery paradigms to be efficiently pursued is a focus of many pharmaceutical companies’ activities.
Traditional electrophysiology platforms: a self-fulfilling prophecy in limiting effective ion channel drug discovery
Ion channels are regulated by both trans-membrane cation and anion gradients and dynamic changes in membrane potential and generate current as a useful index of their activity. As such, the measurement of ionic currents in native tissue or mammalian cell lines using the manual whole-cell voltage-clamp technique has remained the gold-standard measure of ion channel function. While not a focus of this article, other technologies (including radio ligand receptor binding, ion flux and optically-based screening systems) have been employed with varying degrees of success. However, there has been limited success in reproducing the high fidelity, functional data that is generated by measuring the electrophysiological activity of an ion channel in a whole-cell setting. Although automated optical systems have been of general benefit to screening, they have limitations including their sensitivity, the inability to apply multiple protocols such that details regarding rate and state dependence of inhibition cannot be obtained, and the potential for false positives due to direct dye-compound interactions. In addition, there is a relatively long delay between activation of voltage or ligand-gated ion channels and re-equilibration of a membrane potential sensitive dye reflecting such events. In spite of the respectably high throughput of automated optical systems, this can limit their usefulness for investigating some ion channel targets that cycle between several states within a few milliseconds. This is in direct contrast with patch clamping which can resolve such events with much greater temporal resolution.
While the manual whole-cell voltage-clamp technique generates high-fidelity data with excellent temporal resolution it has a number of significant disadvantages that have prejudiced its routine use in a drug discovery environment:
- Throughput is relatively low (serial process allowing <10 compounds to be tested per week)
- Data is only generated when a relatively skilled and highly trained operator is present
- The hardware is relatively expensive
Consequently, few companies have been willing or able to support ion channel drug discovery programs (that typically require 100s of compounds to be screened in a relatively short time frame to generate key structure-activity relationships that drive discovery chemistry efforts) using this manual platform as a primary screening tool. The failure or reluctance of pharmaceutical companies to generate the next generation of ion channel modulators has, in part, therefore been a self-fulfilling prophecy. The inability to screen compounds with sufficient speed and fidelity has dictated that focused ion channel chemical libraries have not been generated and consequently there has been a dearth of novel chemical matter to kick-start new ion channel discovery programs or to investigate the physiological role of ion channels in disease states. In addition, ion channel drug discovery has generally been perceived as relatively difficult, particularly when ‘easier’ targets with high throughput and reliable screening platforms (e.g. automated optical systems) have been available to support them.
In contrast to automated optical screening platforms, the ability of the manual patch clamp technique to discern the affinity of compounds for various ion channel states (e.g. open, closed, inactivated) has added to its gold-standard status. In some ways, this has proved to be somewhat of a burden and raised expectations in that other (non-electrophysiological) higher throughput techniques that have been developed to supersede manual patch have been unable to match the high fidelity of patch data, with investigators reverting to a requirement for patch data to support their ion channel discovery programs.
Moving beyond manual electrophysiology platforms
Over the last decade, it has become increasingly apparent that a new generation of relatively high throughput electrophysiology screening platforms with the ability to generate high fidelity data describing the pharmacology of compounds under physiological conditions needs to be developed and introduced into the pharmaceutical industry to help realise the full potential of ion channels as a drugable target class (Figure 1). There are clear drivers for enhancing electrophysiological screening throughput:
- The ability to appropriately support more ion channel discovery programs
- Attrite compounds earlier and smarter
- Obtain more detailed structure-activity information earlier
- The ability to provide key ion channel-related safety and selectivity data
- Efficient usage of employee time
- Enhance confidence in the ‘do-ability’ of ion channel projects
In response to this, a number of vendors (both established and new) have made considerable investments into developing and refining a new generation of high and higher throughput electrophysiology platforms.
Automating a manually-intensive process
Anyone who has seen or operated a manual patch-clamp setup will appreciate the laborious process involved in generating data from a single cell – one cell at a time. All the steps involved from cell selection, patching onto the surface of the cell, rupturing the cell membrane to achieve the ‘whole-cell configuration’, solution changes and protocol initiation require continual human intervention with many failures during each stage of the process likely throughout an experimental day. In addition, data is only acquired when the investigator is present.
A key development that has enabled this manually intensive process to be automated has been a fundamental change in the way that the whole-cell configuration is achieved. Early systems attempted to replicate the procedures used on manual systems, using image analysis to identify single cells and to direct a prefabricated patch pipette towards the cell. Unsurprisingly, the complex nature of visual cell identification, pipette location and obtaining the whole-cell configuration using this type of automation failed, proving too unreliable and only operating in serial mode.
A number of key changes have helped electrophysiology platforms move beyond serial data collection. Firstly, image analysis is no longer used to locate and identify a single cell. Secondly, the traditional pre-fabricated glass micropipette has been replaced by a planar cassette or electrode chip that permits parallel recordings to be made from multiple cells. These planar electrodes can be manufactured in bulk within tight tolerances, using laser drilling or other etching techniques to fabricate sites or ‘holes’ on which parallel whole-cell recordings from multiple cells can be made. The shift from single serial recordings to parallel recordings from multiple sites has provided a means for a significant increase in throughput. These disposable ‘chip’ format electrodes with tightly regulated resistances form the major consumable of an automated patch system and fit uniquely into their corresponding propriety screening platforms. As such they are constructed in a number of different formats, including from single hole chips (that can be exchanged independently in a platform that uses a number of single chips in parallel), and 16, 384 and 1536 well chips (Figure 2). Each of these platforms utilises a similar but distinct mechanism to bring the cell to the ‘pipette’ (rather than the pipette to the cell in the case of manual systems) to achieve a suitable recording configuration. On the majority of platforms, the traditional whole-cell configuration is achieved and continuous voltage-control is maintained throughout the experimental time course. However, in one of the higher throughput systems available, the amphotericin-mediated perforated-patch configuration is utilised and recordings from a 384 well plate are made in parallel from 48 cells at a time. Each system has a relatively small footprint, occupying less space than a single manual patch clamp system and, since they have been designed to be ‘turnkey’ systems, they do not require a highly skilled electrophysiologist to be operated and require limited training. The majority of these systems use a vacuum to navigate a single cell over a laser-drilled aperture on a disposable ‘chip’ and robotics intelligently deliver compounds. As such, there are no major moving parts requiring operator intervention to achieve the whole-cell recording configuration and to acquire data. Notably, the need for a microscope, air table, faraday cage, electrode puller, micromanipulator and perfusion system is eliminated.
Are these platforms truly high throughput?
The perception of increased throughput is often based upon prior experience. For example, the robotic and analytical infrastructure to automate and maximise the throughput of binding and enzymatic assays is widely used. As such, it is unlikely that an additional increase in throughput would have a significant impact on drug discovery programs directed at these target classes. In contrast, manual ion channel electrophysiology has always been at the low end of low-throughput assay platforms and as such even a 10-fold increase in throughput would have a major effect on the ability to support project progression. So, in comparison to ultra-high throughput platforms, which can screen tens of thousands of compounds per week, the first generation of ‘high’ throughput systems may still appear as relatively low throughput. However, in the ion channel arena they represent a significant improvement and enhancement in the ability to prosecute ion channels as drug targets.
Key considerations when implementing high-throughput patch platforms
The rationale to support the introduction of new technologies is often based on cost versus benefit. Cost encompasses not only the purchase of the hardware but also support and servicing, training, consumables, IT support, employee costs and so on. Similarly, benefit is a multi-faceted equation, including throughput, employee requirements, short and long-term impact on discovery goals, colleague career development and so on. In an environment where the number of ‘easy’ drug targets is declining, the need to build the infrastructure to enable the potential of some of the traditionally more ‘difficult’ target classes to be realised has increased. These new technological advancements have helped shift the cost-benefit ratio in favour of bringing more ion channel targets into drug discovery portfolios, in addition to expanding the ability to address ion channel-related cardiovascular safety issues that form a part of every drug discovery program. Ion channel targets are increasing being perceived as more ‘do-able’, based upon an ability to rapidly generate high quality screening data, in sufficient quantities to support rational drug design.
An important and often labour-intensive aspect of introducing automated electrophysiology platforms is their optimisation and validation. Often, data has been historically generated on a manual electrophysiology system and, particularly in the case of hERG measurements, key experiments need to be designed and performed to compare the pharmacology of a panel of reference compounds on both platforms. While the principle of measuring compound efficacy is essentially the same on both platforms, there are key differences that may alter apparent pharmacology. These include:
- Compound absorption/retention on the planar electrode substrate and 96-well plates containing test compounds
- Compound addition rate to the test well
- Compound loss via absorption to excess cells in each well
- Test protocols
System validation
Many companies introducing automated patch clamp systems are doing so to replace or compliment existing manual patch clamp resources that have been used historically to generate hERG safety data or limited screening and selectivity data. It is critical, therefore, to undertake careful validation experiments to compare data on reference compound sets obtained on both platforms prior to endorsing their routine use. These studies should focus on a number of criteria that may potentially alter compound pharmacology. These include:
- The influence of temperature since the automated systems operate solely at room temperature
- The type of vessel used for compound preparation and storage
- Rate and number of compound additions
- The potential for the large numbers of cells per chip well to act as a drug sink, particularly for lipophilic compounds.
Apart from temperature, each of these variables has been found to have an impact on compound pharmacology. As a result, it is now commonplace to use glass vessels to prepare stock compound solutions and glass-bottomed 96-well plates to store compound dilutions on the automated patch clamp system to limit compound absorption. In addition, multiple sequential drug additions at a single concentration may be used to ensure effective drug delivery at the site of action. Combining these experimental changes has ensured that there is good agreement between compound potencies on manual and automated patch platforms (Figure 3).
Cell lines
One prerequisite for maximising the throughput of electrophysiological systems is the generation and selection of clonal cell lines expressing the ion channel target of interest and their optimisation for use on the screening platform being employed. In the past, the generation and characterisation of proprietary cell lines has not been a trivial matter, relying on pre-screening with optical systems and/or low-throughput manual patch clamp platforms to serially test a small number of cells from a small number of dilution clones. As such, cell lines have often not been truly optimised as decisions have been made based upon a small amount of data from a small number of dilution clones. A significant benefit of the new high throughput platforms is the ability to allow rapid follow-up testing of flow-cytometer sorted cell lines. This allows robust cell lines to be selected on the basis of a large dataset from a large number of clones describing:
- Current amplitude
- Frequency of expression
- Success of sealing
- Longevity of the recording period available
A range of cell backgrounds are suitable for use on automated platforms including HEK, CHO, CHL and RBL. In many cases, however, CHO cell lines have proven to perform best.
Paradoxically, automated patch clamping has created a need for cell lines with specific requirements but at the same time it offers a quick way to screen for them.
Cell line husbandry is also a key aspect of optimising the performance of the automated patch systems. Harvesting cells during their logarithmic growth phase with tightly regulated trypsinisation or other cell harvesting procedures, in addition to carefully controlled tituration of cells both on and off the recording platform are key to maximising success. Increased throughput using automated patch clamp systems has a large impact on cell husbandry support. Operating a manual patch clamp system only requires a single T25 flask of a particular cell line per experimental day. In contrast, each experimental run using an automated system requires at least a single T25 flask of cells. Over an experimental week this may translate into more than a five-fold increase in cell usage. Additionally, if a number of different cell lines are being simultaneously used then this may impose an additional burden on cell culture resources.
Don’t get rid of your manual patch systems
In a rational screening paradigm, a number of different experimental models are commonly employed to gather useful structure-activity relationship data. Each provides a balance between information content and throughput and, as such, each has a particular value. One commonly used philosophy used to design screening funnels is to select the appropriate experimental tool to address the question being asked, rather than attempting to use a single assay platform to answer all questions. In many cases, automated patch clamp systems may provide an appropriate balance between throughput and data quality to support the identification and routine characterisation of new chemical matter. However, to answer more exquisite questions regarding mechanism of block, affinity for a particular channel state, rate-dependence of inhibition and compound on/off rate the manual patch clamp system (or an alternate automated patch clamp system) may still be the platform of choice, despite its low throughput. This is since usually only a limited number of these studies are performed on compounds of particular interest whose primary pharmacology had already been established on an automated platform. Manual patch clamp systems still offer an advantage in that protocols may be changed and adapted ‘on the fly’. In addition, they allow experiments to be performed using both freshly dissociated cells from native tissue and clonal cell lines. Consumable costs for automated platforms are still relatively high ($100-150 per chip) and so cost-efficiency may focus their use on high throughput compound screening (Table 1).
Summary
Automated patch-clamp techniques allow researchers to employ direct electrophysiological determination of compound activity on channels, on much larger numbers of compounds and at much earlier stages of the drug discovery process than with earlier tools and techniques. This is helping accelerate lead identification and optimisation stages of the drug discovery process in addition to aiding the expansion of selectivity testing and the addition of more ion channel targets to discovery portfolios. Whereas in the past, researchers relied on low fidelity assays or used multiple skilled electrophysiologists on a single project to drive drug discovery, now a single electrophysiologist can support multiple projects, providing more data more rapidly.
The integration of automated, high(er) throughput electrophysiology platforms is now gaining pace throughout the pharmaceutical industry and heralds a new golden age of ion channel research and drug discovery.
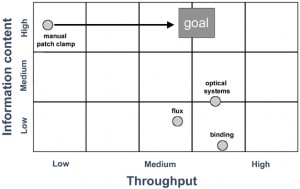
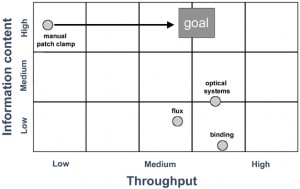
Figure 1: Traditional ion channel screening platforms have either focused on throughput (flux, binding, optical systems) or information content (manual patch clamp). To support drug discovery programs, new ion channel screening platforms that are able to provide high fidelity data with high throughput are required
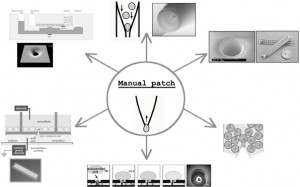
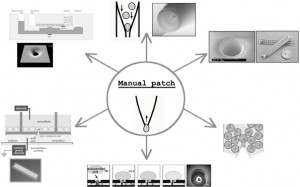
Figure 2: A number of unique approaches have been utilised by vendors to develop alternative methods to the manual patch system to make parallel electrophysiological recordings from multiple cells. Most use a disposable planar electrode format assembled as a ‘chip’ or packaged as single units. Each disposable fits the vendor’s proprietary screening platform
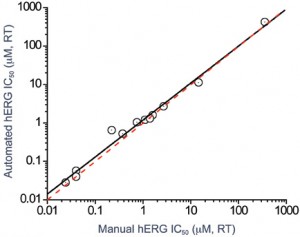
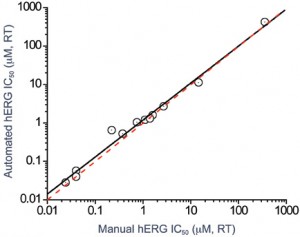
Figure 3: Correlation between hERG potency data for a range of reference compounds obtained using a manual patch-clamp system and an automated ion channel-screening platform. The potency of the compounds was essentially identical on each platform following assay optimisation studies (involving the use of glass compound preparation vessels, glass compound plates and multiple drug additions)