How implantable systems and microparticle depot injections are meeting the needs of long-acting drug delivery
Posted: 26 June 2020 | Jite Okoh (LLS Health), Kimberly Zubris (LLS Health) | No comments yet
Implantable systems and microparticle depots are used in many areas of drug delivery to release medicines at a controlled rate within the body. The use of these long-acting dosage forms eliminates the need for frequent administrations via traditional routes of administration, which ensures patient compliance and results in improved outcomes. Dr Kimberly Zubris and Jite Okoh discuss the benefits these long-acting products bring to drug delivery and address the critical design, manufacturing and testing challenges that should be considered in their development.
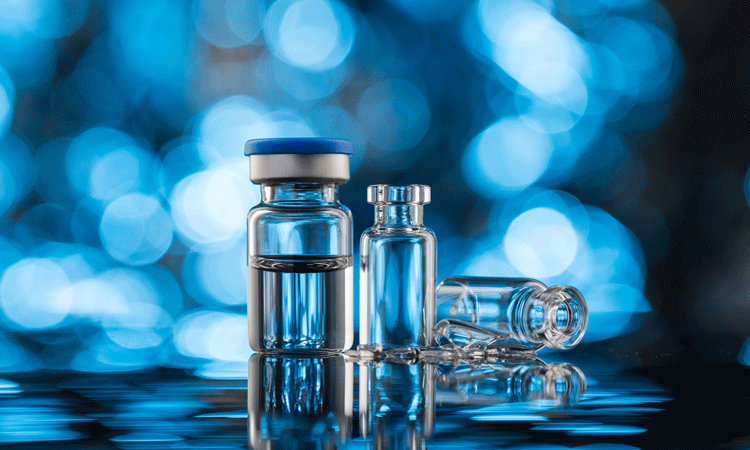
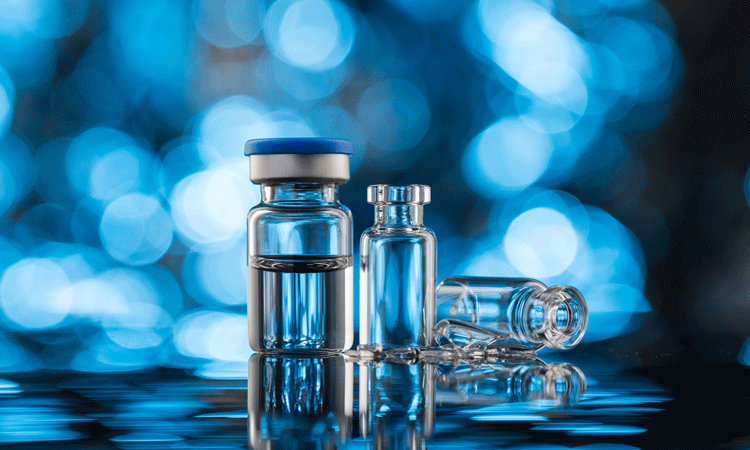
The advantages of implantable systems and microparticle depot injections
Drug delivery systems based on implants and depots allow for controlled and long-lasting delivery of active pharmaceutical ingredients (APIs) in the body, which leads to multiple patient benefits. Long-acting dosage forms release API over weeks, months or even years with a single injection or implantation. This replaces the frequent dosing associated with traditional pills or injections and reduces the burden of compliance on patients. Delivering drugs directly to a local environment or to the bloodstream also avoids gastric and hepatic degradation of the API. This enables drug products that use less API to achieve the same therapeutic effect and potentially have reduced side effects.
In addition to the numerous patient benefits of long-acting drug products, there are attractive commercial incentives; pharmaceutical companies can benefit from the high revenue potential of novel dosage forms, which may have intellectual property protection and offer significant differentiation. Long-acting dosage forms also serve as a lifecycle management tool for APIs at the end of their patent lives. By reformulating known APIs into long-acting implants or depot injections, pharmaceutical companies can create value and offer a better product for patients.
Marketed long-acting drug products
Implants available today can be divided into two categories: biodurable and bioresorbable. Biodurable polymers do not degrade or reabsorb into the body and are delivered via several routes of administration. Examples of biodurable implants include intravaginal rings (used in contraception, hormone replacement therapy and cancer), intraocular implants (for diabetic macular oedema or uveitis) and subcutaneous implants (with uses in contraception, diabetes and opioid addiction). Biodurable systems typically release drugs by Fickian diffusion, wherein a drug molecule diffuses through a polymer until it reaches the interface between the implant and tissue where it is absorbed. Biodurable implants deliver drugs for weeks to years and remain intact in the body, enabling removal when treatment is completed or no longer desired.
Bioresorbable polymers degrade in vivo to release a drug at the site of implantation. Examples of bioresorbable implants include intrathecal wafers that are placed in the brain following the removal of a glioma and intraocular implants that treat glaucoma and age-related macular degeneration (AMD). Bioresorbable systems often degrade completely over weeks to months as they release a drug, so surgical removal is not required.
Microparticle depot injections made up of bioresorbable polymers are also proving to be successful commercial drug products. They are used to treat a range of indications – such as cancers, central nervous system disorders and more – and can be delivered by different parenteral routes of administration, including intramuscular, subcutaneous, intravitreal and periodontal. Depot injections typically have a duration of action and dosing frequency of two weeks to six months, during which they completely degrade.
Implantable systems
Challenges in the development of long-acting implants fall into three main categories: design, manufacturing and in vitro testing.
Design challenges
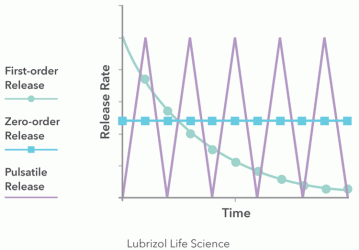
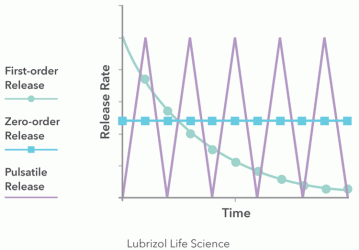
Figure 1: Various types of drug release profiles
The first consideration in designing implants is whether a monolithic matrix or a reservoir system should be used. This decision determines the rate at which a drug will be released. To identify the most suitable design, the desired drug release kinetics need to be understood (Figure 1). A reservoir implant design, which consists of a drug-loaded core surrounded by a rate-controlling polymer sheath, is appropriate if a constant release rate (ie, zero-order release kinetics) is required at the site of implantation. If the rate of drug release into the body is not so critical and an initial burst of API (ie, first-order release kinetics) is desired or tolerable, then a matrix implant can be selected. Matrix implants simply consist of drug homogenously dispersed in a polymer.
Dimensions also need to be considered during this phase, as the implant’s surface area can strongly affect release rate. For matrix-based, rod-shaped implants, a larger diameter results in a higher surface area and faster drug release rate. If a reservoir design is used, the rate-controlling membrane thickness is also an important factor.
A further consideration during design is drug loading. An implant must contain enough of the drug to provide the desired therapeutic effect but increasing drug loading also increases the release rate in a non-linear fashion, making the impact challenging to predict. Therefore, hands-on investigation or modelling of the relationship between drug loading on release rate is critical to achieving the desired drug release in vitro and in vivo.
Polymer chemistry has a significant impact on the performance of both biodurable and bioresorbable implants. Selecting polymers that are compatible with specific APIs or degrade at the desired rate are just some of the factors to consider. However, all polymers used in implantable drug delivery systems should be from current good manufacturing practice (cGMP)-approved sources. They also must be biocompatible and meet ISO 10993 and USP <1031> requirements. Furthermore, if they are for prolonged mucosal contact, polymers will also need to meet the USP class VI standard.
Manufacturing challenges
One of the first considerations when manufacturing implantable systems should be how an API will be mixed into the polymer. This can be done by dissolving the polymer and API in a common solvent; however, for large-scale development, hot melt extrusion presents the most appropriate drug incorporation technique. Once mixed, the extrusion process can be used to produce matrix implants in the shape of rods, films or tubes. Alternatively, injection moulding can be used for more complex shapes.
By reformulating known APIs into long-acting implants or depot injections, pharmaceutical companies can create value and offer a better product for patients”
A common reservoir implant design is the core-sheath system, wherein a drug-loaded polymer is surrounded by a second polymer acting as a rate-controlling membrane. The manufacture of core-sheath implants can be achieved through co-extrusion, which is a continuous process using multiple extruders.
In situations where only small amounts of polymer or API are available, solution casting or powder blending of the API and polymer enable small-scale prototyping. Blended powders can be processed in a mini twin screw extruder or vacuum compression moulder with small batch sizes. A mini injection moulder can also be employed if an injection moulding process is required.
In vitro release testing
In vitro drug release testing (IVRT) measures drug release in a controlled lab environment. These tests must be representative, sensitive and reproducible; therefore, the medium used to suspend the implant for in vitro testing should reflect the in vivo conditions. It is also important to choose the appropriate stirring/soaking procedure, agitation rates and temperature.
When measuring in vitro release testing, sink conditions must be maintained for the entire duration, meaning that the implant is submerged in enough fluid to maintain a concentration gradient and ensure API release is only driven by Fickian diffusion. Proper sampling frequency is also important to build a representative curve of drug release, while testing for the proper duration is vital for simulating in vivo conditions.
IVRT provides a good assessment of the effects of materials and design choices, as well as estimating the timeframe of drug release. It is also useful for predicting long-term release from short-term data, in addition to developing, refining and validating implant models. However, IVRT is limited in predicting in vivo performance, which should instead be performed in an animal or human model. Therefore, it should be used in the design of implant devices to help understand the controlling factors, but testing should move to in vivo as soon as possible.
Microparticle depot injections
Fabricated from API alone or in combination with protective and rate controlling materials such as polymers, microparticles may take the form of matrix-like microspheres or microcapsules that have a distinct core/shell structure. Depots most often consist of solid polymeric microspheres, physically produced with biodegradable polymers. Once formulated these microparticles are often lyophilised or freeze dried to generate stable dry powders, which are maintained in a dry state until they are combined with a diluent prior to administration. As depots are parenteral or injectable, they must be supplied in sterile form, requiring either terminal sterilisation or aseptic manufacturing techniques. Once injected, depot formulations degrade over timeframes that typically span weeks to months.
Design challenges
While microparticles have several advantages, they also share many of the formulation challenges associated with implants. However, different release profiles can be achieved by adjusting polymer selection, particle size and drug loading. When preparing depots from bioresorbable polymers, the exact polymer chemistry and molecular weight must be explored and optimised. Formulators should work closely with their analytical counterparts to ensure polymer and API compatibility and to identify a sterilisation process that will not negatively impact the properties of either.
Depot injections typically have a duration of action and dosing frequency of two weeks to six months,
during which they completely degrade”
Microparticle depots are also subject to some unique considerations. Particle size distribution (PSD) can have a major impact on performance; if the particles in a depot injection are polydisperse, meaning there are a wide range of particle sizes within the formulation, product performance will be inconsistent from dose to dose. Ideally, microparticles are monodisperse, meaning they have a narrow particle size distribution, which minimises variation between injections and/or batches of product.
Mean size is also critical when targeting a monodisperse PSD. If particles are too small, they can be susceptible to phagocytosis and may be metabolised prior to achieving their therapeutic effect. If they are too large, the particles will be difficult to administer through a syringe. Finding the right PSD is achieved with formulation expertise and process optimisation aided by design of experiments (DoE).
Manufacturing challenges
The most common approaches to manufacturing microparticles are emulsification and solvent extraction or evaporation. A typical emulsification process will utilise high-shear mixing to prepare the initial emulsion phase from which the solvent is removed via extraction, evaporation or a combination of both. This technique often produces a broad PSD requiring further downstream processing and size classification. It also tends to result in a lower product yield. Membrane emulsification systems, microporous and microfluidic systems are useful – and potentially more efficient – approaches to prepare microparticle depots.
Maintaining particle size pre- and post-lyophilisation is also challenging and a well-designed formulation containing the proper lyoprotectants can help. These are often sugars that immobilise microparticles to protect them from agglomerating or being affected by the stresses of freezing and drying. A further challenge in the preparation of microparticles is the concern with residual solvents. During microparticle depot production, solvent systems are utilised to solubilise the polymer and/or the API during processing. The International Council for Harmonisation of Technical Requirements for Pharmaceuticals for Human Use (ICH) guidelines outline the acceptable amounts for residual solvents in pharmaceuticals to ensure patient safety. The Q3C (R7) guideline specifically recommends the use of less toxic solvents and provides the limits that are toxicologically acceptable for others.
In vitro release testing
As noted with implants, IVRT testing methods with good discriminatory ability are critical for quality control purposes. While methods that can be used in the development of in vitro and in vivo correlation are often required for complex parenteral products like microparticle depots, it is important to understand that these tests are most useful for assessing material and design choices.
Conclusion
Long-acting implants and microparticle depot injections offer many advantages and product development opportunities. However, choosing the proper design, materials and manufacturing techniques to create a long-acting drug product are not trivial tasks and must be executed properly.
About the authors
Issue
Related topics
Drug Delivery Systems, Drug Manufacturing, Drug Safety, Manufacturing, QA/QC
Related organisations
Related diseases & conditions
age-related macular degeneration (AMD), Cancer, Diabetes, diabetic macular oedema, Glaucoma, glioma, uveitis