Promising biocompatible and biodegradable drug nanocarriers for cancer treatment and bone defect repair
Posted: 17 February 2021 | Tsun-Kong (TK) Sham, Ying-Jie Zhu | No comments yet
Drug nanocarriers based on calcium phosphates and calcium silicates have attracted much interest in recent years owing to their excellent biocompatibility and biodegradability, high drug loading capacity, sustained and targeted drug delivery and promising applications in cancer therapy and bone defect repair. However, the research on drug-carrier interactions is a significant challenge. Herein, we introduce our recent studies on tracking drug-nanocarrier interactions by X-ray absorption near edge structure and X-ray spectromicroscopy using synchrotron radiation.
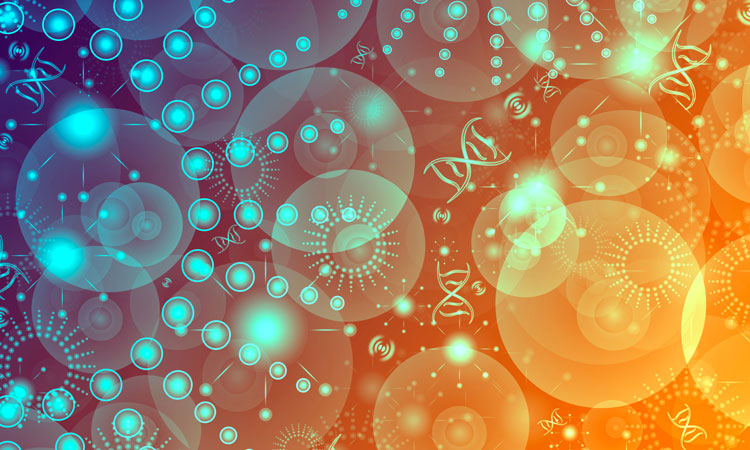
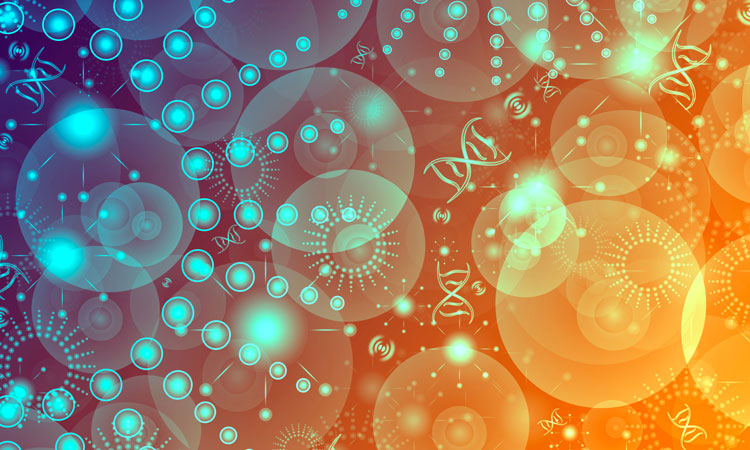
The advent of synchrotron-based techniques provides unprecedented capability for tracking and analysing drug-carrier interactions at the molecular level. X-ray absorption near-edge spectroscopy (XANES) is an element- and site-specific core-level absorption spectroscopic technique, which can reveal the chemical environment of the absorbing atom. In addition, scanning transmission X-ray microscopy (STXM) with a nanoscale beam can record XANES of an element in a desired region of interest and image an individual drug carrier in the transmission mode, pixel by pixel in its entirety with a pixel size of ≤30µm×30µm. Synchrotron, an oval electron accelerator-based light source, provides the intense, highly collimated and energy tunable X-rays required for these studies.
We have been using XANES and STXM to track and analyse the drug-nanocarrier interactions in the above-mentioned systems before, during drug uptake and release and after drug release at the molecular level.7-12 We found that Ca2+ ions from calcium silicate hydrate (CSH) nanocarriers interact with the carboxyl groups of the ibuprofen (IBU) drug molecules,7 hence an esterification‑like interaction between silanol groups (Si-OH) on the CSH nanocarrier surface and carboxylic groups of IBU is established. We also investigated drug‑nanocarrier interactions using two other drugs, alendronate sodium and gentamicin sulfate.8 Clearly discernible spectral differences were apparent at the Ca and Si K-edge XANES of the CSH nanocarrier before and after loading for these drugs, indicating that drug molecules were linked to Ca-OH groups of the nanocarrier primarily by electrostatic interaction. In addition, silanol (Si‑OH) groups on the CSH nanocarrier surface also provide active sites for interactions between the CSH nanocarrier and drug molecules. Compared with alendronate sodium and gentamicin sulfate, the CSH exhibits an extremely high IBU drug loading capacity, indicating that the functional groups of drug molecules could significantly influence the drug loading capacity.8
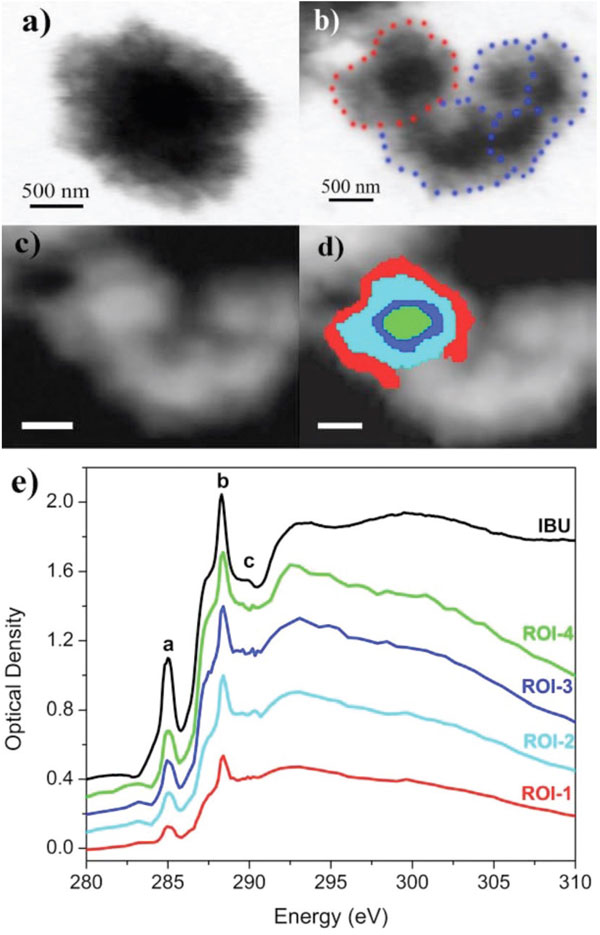
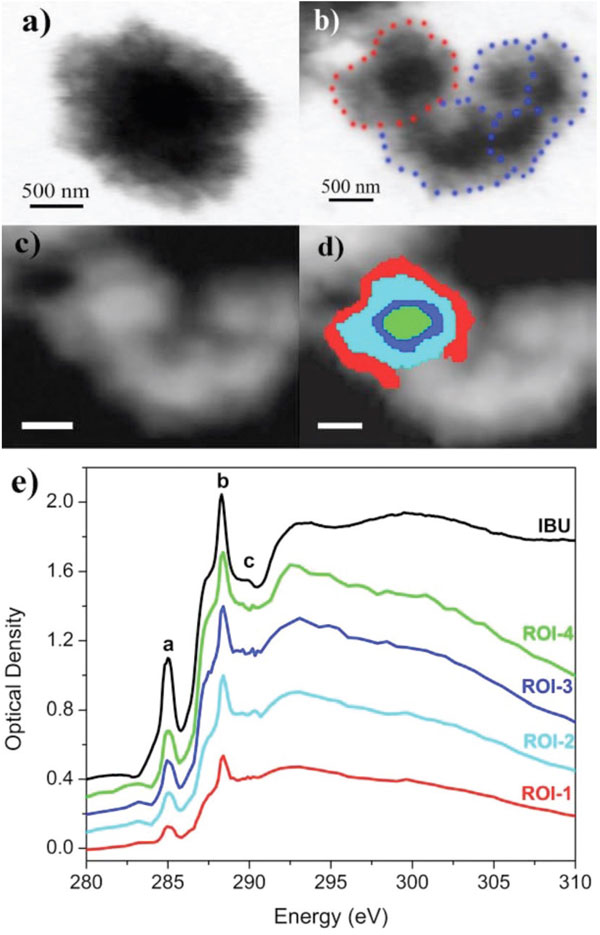
Figure 1: STXM images of an individual CSH microsphere: (a) before and (b) after IBU loading taken at the Ca L3,2-edge (E=352.5eV); (c) STXM image of an individual CSH microsphere; (d) different regions of interest (ROIs) taken from an individual CSH microsphere; red: ROI-1, cyan: ROI-2, blue: ROI-3, green: ROI-4. Scale bars in (c) and (d) are 500nm; (e) XANES spectra from each ROI displayed in (d) at the C K-edge. Reproduced from Guo XX, et al.9
Furthermore, we used STXM combined with XANES to image an individual CSH mesoporous microsphere loaded with IBU (Figure 1). We found that the C K-edge XANES obtained from different nanoscale ROIs exhibit identical features. Compared with the spectrum of the powdered IBU (Figure 1e) there is no change in spectral features, confirming that the IBU molecules remain intact after loading.9
We can also obtain the drug distribution in an individual CSH mesoporous microsphere (Figure 2). From Figure 2a, we see that after drug loading, the thickness of the central area of a CSH mesoporous microsphere (Si absorption) is ~160nm and that of the peripheral surface is ~18nm. The thickness distribution mapping of IBU (C absorption) is shown in Figure 2b where the carbon signal is only from IBU molecules. The amount of loaded drug in the drug nanocarrier can be estimated quantitatively from the thickness of CSH and IBU. It is apparent from Figure 2 that IBU molecules are more abundant in the thick area (IBU thickness: ~26nm) compared to the peripheral surface (IBU thickness: ~2-3nm). The thickness ratio of Si to C (representing the thickness ratio of CSH to IBU) is the same in different regions, indicating that IBU molecules are uniformly loaded into CSH mesoporous microspheres.9
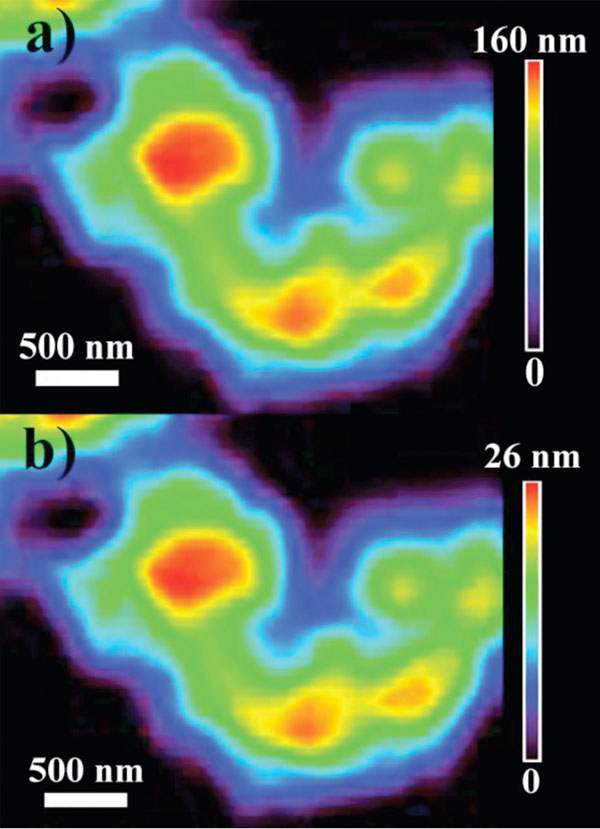
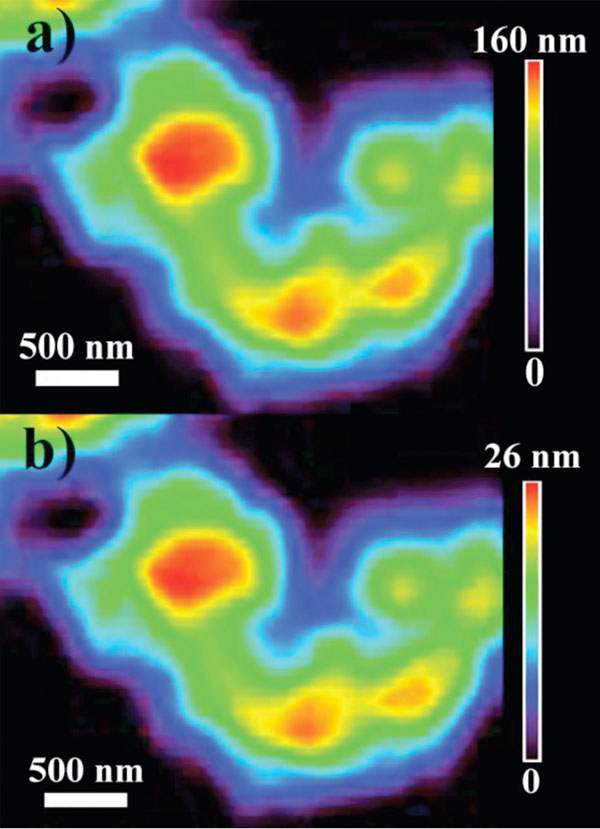
Figure 2: Thickness distribution mappings of an individual CSH mesoporous microsphere after IBU loading at (a) Si K-edge and (b) C K-edge. The vertical bar shows the colour code of the material thickness. Reproduced from Guo XX.9
In addition to revealing drug-nanocarrier interaction, we have investigated the biomineralisation mechanism of CSH mesoporous microspheres during drug release in simulated body fluid using XANES and STXM.10 We clarified the presence of amorphous CaCO3 at the early stage of biomineralisation, which was still controversial prior to this work. It was found that amorphous SiO2 from the hydrolysis of silicate ions could provide preferential sites for the aggregation of hydroxyapatite, a harmless component to the body.10
In another study using calcium phosphate as a nanocarrier, we found that adenosine 5’-triphosphate (ATP) biomolecules played an important role in the formation of amorphous calcium phosphate nanocomposite (ACP), which is an ideal drug nanocarrier owing to its high biocompatibility and excellent biodegradability. We used the XANES together with STXM to reveal the interactions of ATP and drug molecules with individual ACP mesoporous nanocomposites.12 It was found that the adenosine and phosphate groups of ATP biomolecules could co-ordinate with Ca2+ ions and played critical roles in the formation of ACP mesoporous nanocomposite. Subsequently, we tracked the interaction of the ACP carrier with haemoglobin and discovered that haemoglobin’s –COOH groups were linked to Ca2+ ions primarily by electrostatic interaction and the N-containing ring structure within the drug molecules also co-ordinated with Ca2+ ions. In addition, hydrogen bonds formed between –OH or –NH2 groups of drug molecules and PO43– groups of the nanocarrier.12
Our studies have demonstrated that the synchrotron radiation-based XANES and STXM are powerful techniques to help understand the carrier‑drug interactions in drug delivery systems, which in turn provides feedback to nanocarrier design. These findings provide insights and guidance for further surface modification or functionalisation of drug nanocarriers to enhance the drug loading capacity, control the drug release kinetics and open new possibilities for biomedical applications. In addition, it is also desirable to adopt another synchrotron technique, X-ray-excited optical luminescence (XEOL), to track the behaviour of rare earth ions-doped drug nanocarriers using rare earth ions as an “X-ray in, visible light emission out” probe for application in bio-imaging.13 We highly anticipate that nanostructured calcium phosphates and calcium silicates-based drug delivery systems will lead to novel high-biosafety nanoplatforms for various clinical applications such as cancer treatment and bone defect repair.
Ying-Jie obtained his PhD from the University of Science and Technology of China in 1994. He worked as a Visiting Scholar at the University of Western Ontario, Canada, Alexander von Humboldt Research Fellow at the Fritz-Haber Institute, Germany and Postdoctoral Fellow at the University of Utah and University of Delaware, US, from 1997 to 2002. Then, he became a full professor at Shanghai Institute of Ceramics, Chinese Academy of Sciences. He has published about 370 peer-reviewed journal papers and three book chapters and has more than 60 patents granted. His main research interest focuses on nanostructured biomaterials.
TK is a Distinguished University Professor at Western University. He obtained his PhD from Western, then returned to his alma mater in 1988 after a decade on the staff of Brookhaven National Laboratory. He was the Director of the Canadian Synchrotron Radiation Facility at the Synchrotron Radiation Center in Stoughton Wisconsin, a founding member of the Canadian Light Source (CLS), a national facility of advanced photon technology in Saskatoon commissioned in 2005. He is presently a Tier I Canada Research Chair (2002-2023) and the Beam-team Leader of three beamlines at the CLS. His research interest is the interplay of materials and synchrotron radiation.
References
1. Cai AY, Zhu YJ, Qi C. Biodegradable inorganic nanostructured biomaterials for drug delivery. Adv Mater Interfaces. 2020; 7(20):2000819.
2. Chen F, Zhu YJ. Multifunctional calcium phosphate nanostructured materials and biomedical applications. Curr Nanosci. 2014; 10(4):465–85.
3. Zhu YJ, Chen F. pH-responsive drug delivery systems. Chem Asian J. 2015; 10(2):284–305.
4. Qi C, Musetti S, Fu LH, Zhu YJ, Huang L. Biomolecule-assisted green synthesis of nanostructured calcium phosphates and their biomedical applications. Chem Soc Rev. 2019; 48(10):2698–737.
5. Zhu YJ, Sham TK. The potential of calcium silicate hydrate as a carrier of ibuprofen. Expert Opin Drug Deliv. 2014; 11(9):1337–42.
6. Zhu YJ, Guo XX, Sham TK. Calcium silicate-based drug delivery systems. Expert Opin Drug Deliv. 2017; 14(2):215–28.
7. Guo XX, Wu J, Yiu YM, Hu YF, Zhu YJ, Sham TK. Drug-nanocarrier interaction-tracking the local structure of calcium silicate upon ibuprofen loading with X-ray absorption near edge structure (XANES). Phys Chem Chem Phys. 2013; 15(36):15033–040.
8. Guo XX, Wang ZQ, Wu J, Yiu YM, Hu YF, Zhu YJ, et al. Tracking drug loading capacities of calcium silicate hydrate carrier: a comparative X-ray absorption near edge structures study.
J Phys Chem B. 2015; 119(31):10052–59.
9. Guo XX, Wang ZQ, Wu J, Wang J, Zhu YJ, Sham TK. Imaging of drug loading distributions in individual microspheres of calcium silicate hydrate – an X-ray spectromicroscopy study. Nanoscale. 2015; 7(15):6767–73.
10. Guo XX, Wang ZQ, Wu J, Hu YF, Wang J, Zhu YJ, et al. Tracking the transformations of mesoporous microspheres of calcium silicate hydrate at the nanoscale upon ibuprofen release: a XANES and STXM study. CrystEngComm. 2015; 17(22):4117–24.
11. Guo XX, Wu J, Yiu YM, Hu YF, Zhu YJ, Sham TK. Effects of polymer intercalation in calcium silicate hydrates on drug loading capacities and drug release kinetics: An X-ray absorption near edge structure study. Can J Chem. 2017; 95(11):1122–9.
12. Jiang YY, Wang ZQ, Chen JT, Li J, Zhu YJ, Liu LJ, et al. Tracking the interaction of drug molecules with individual mesoporous amorphous calcium phosphate/ATP nanocomposites – an X-ray spectromicroscopic study. Phys Chem Chem Phys. 2020; 22(23):13108–17.
13. Wang Z, Wang J, Hou D, Sham TK. Imaging of individual Eu doped Y2O3 sub-microspheres using photoluminescence yield: an application of scanning transmission X-ray microscopy in luminescent materials. Microsc. Microanal. 2018; 24(S2):480–1.
Issue
Related topics
Drug Delivery Systems, Drug Development, Nano-medicine, Technology