The imperative of fridge-free vaccines
Posted: 19 December 2023 | Bruce Roser (Stablepharma ) | No comments yet
Bruce Roser of Stablepharma gives an overview of efforts to develop thermostable vaccines. Could ‘fridge-free’ formulations improve the cost-effectiveness and accessibility of vaccination?
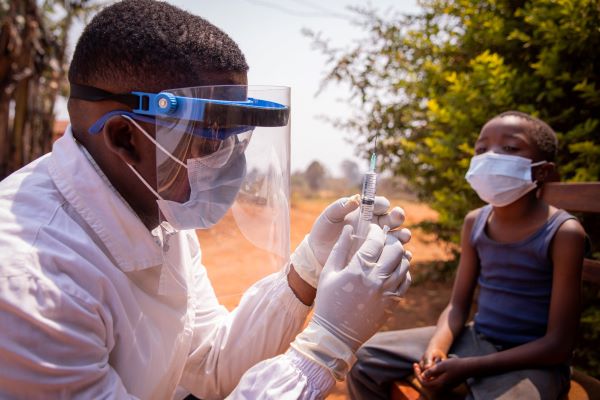
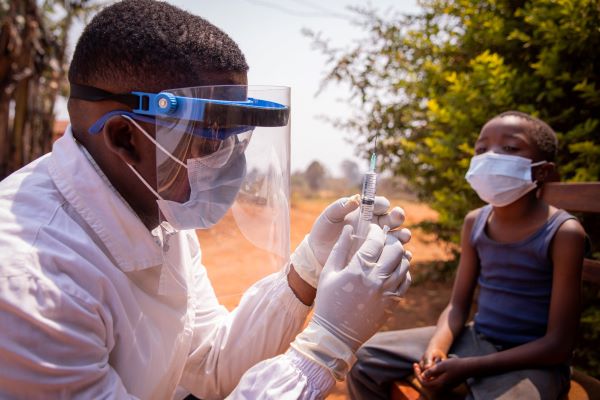
Many of today’s vaccines are produced in ready-to-inject liquid formulations that must be kept cold to maintain stability. A requisite cold chain has been designed and implemented to be uninterrupted from factory to patient. However, this greatly complicates both the worldwide distribution and stockpiling of vaccines and other drugs.
Since only about 20 percent of the world’s population is lucky enough to live in areas where reliable refrigeration is available,1 the remaining majority live with uncertainty regarding access to completely safe and secure vaccines. In fact, breakdowns in correct temperature storage have resulted in about 50 percent of all vaccines produced being inactivated by either overheating2 or by freezing.3
It is generally acknowledged that to protect the population, vaccination must be extended to everyone worldwide. This requires the development of technologies to rapidly produce effective, safe, stable vaccines that can be manufactured and distributed quickly.4-6 Major non-governmental organisations (NGOs) such as Médecins Sans Frontières have long been calling for the development of thermostable vaccines to ease delivery to areas of the world with more challenging conditions.7 The additional substantial economic benefits that flow from room-temperature storability have also been thoroughly documented.8
A wide range of pharmaceuticals including vaccines have been thermally stabilised to comply with this requirement by being dried from solutions of water-soluble glasses, particularly sugar glasses and most effectively trehalose.9-11 All these dry, stabilised actives are unaffected by high-ambient and freezing temperatures.
The mechanisms of trehalose stabilisation
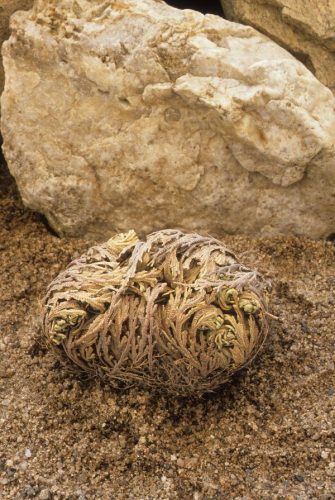
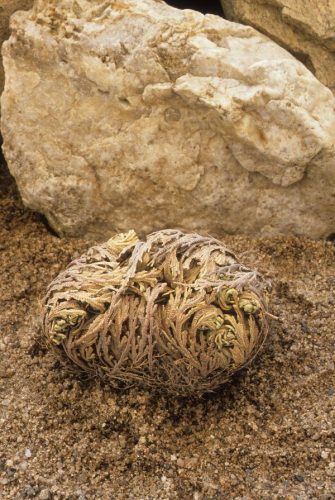
The Resurrection plant was the inspiration for Stablepharma’s stabilisation technology. It can remain completely dry and apparently dead for many years without decay, yet its leaves unroll into a vigorous growing plant when rehydrated. It contains a high concentration of trehalose when dry.
The Resurrection plant was the inspiration for Stablepharma’s stabilisation technology. It can remain completely dry and apparently dead for many years without decay, yet its leaves unroll into a vigorous growing plant when rehydrated. It contains a high concentration of trehalose when dry. Underlying thermal stabilisation by sugars is the ability of drying sugar solutions to undergo glass transformation rather than crystallisation.12 This results in the product being incorporated into the amorphous glassy solid rather than being excluded from a mass of pure crystals, as occurs with other popular excipients such as lactose and mannitol. Dry trehalose glasses are physically very stable, with a glass transition temperature (Tg) of 106°C while sucrose glasses have a Tg of only 60°C.13
Trehalose, again unlike most other sugars, is chemically inert and does not react with other components of the product. It is also very resistant to hydrolysis having a very low glycosidic bond energy of -4.2 kJ/mol, unlike sucrose which in contrast has a high glycosidic bond energy of +113 kJ/mol.14
Sucrose is an excellent glass former and short‑term stabiliser. However, although sucrose is widely thought of as a suitable stable sugar with a spontaneous half-life of hydrolysis at neutral pH of 440 years, at low pH it is very susceptible to hydrolysis (and this reaction is much faster at higher ambient temperatures).
In the presence of proton-donor groups in the product or formulation, sucrose hydrolyses to the monosaccharides glucose and fructose. The reactive aldehyde or ketone groups of these reducing sugars then underdo carbonyl amine reactions with proteins to form Schiff bases, followed by a complex chemical cascade, the Maillard reaction,15 causing progressive chemical degradation unless the dried formulation is kept constantly refrigerated. Obviously, to properly stabilise a drug or vaccine for long-term, room‑temperature storage it is essential to use inherently stable and completely non-reactive excipients that cannot attack the product. Trehalose has a half-life of spontaneous hydrolysis of 6.6 million years, ie, it is 15,000 times more stable than sucrose and its rate of acid hydrolysis is less than the detection limit of one percent at a pH of 3.5 at 100°C for 24 hours.16
Vaccines and other drugs for rapid and safe worldwide distribution should be free from the need for special storage conditions”
Vaccines and other drugs for rapid and safe worldwide distribution should be free from the need for special storage conditions. World Health Organization (WHO) guidelines for “room temperature storable” drugs advise there must be no degradation after at least six months storage at 40°C and 75 percent relative humidity.17 Unfortunately, many of the lyophilised parenteral pharmaceuticals currently referred to as “stable” or “stabilised” after freeze-drying are only stable long-term if stored carefully refrigerated and thus fail this criterion.
New approaches to improve stability of vaccines
More recent attempts to improve the stability and ease of use of vaccines have used techniques such as immobilisation on thin membranes,18 silk fibres,19 and cages of silica.20 Microneedle array patches with the vaccine coating the needles,21 or even within the substance as dissolving needles22 are also being developed. The most obvious problem affecting all of these options is the very small volume of vaccine that can be stabilised, ranging from ~50µl for porous membrane devices to only a few microlitres for microneedle arrays. This restricts use to highly potent vaccines such as living virus vaccines, or nucleic acid-based vaccines such as the mRNA/liquid nanoparticle COVID-19 vaccines.
The use of foreign materials that require removal before injection are also impracticable. Microneedle array patches have ignited much recent interest and enthusiasm as a potentially simple, safe and painless way to vaccinate. However, so far manufacturing has only been achieved on a modest scale. Indeed, in a recent paper describing the vacuum-assisted printing of dissolvable microneedle arrays,22 the authors estimate that “a first-generation printer is capable of manufacturing 100 patches in 48 hours”. Scaling up is necessary and may even be possible, but there is clearly a long way to go to achieve rapid throughput of the many millions of doses of vaccines needed. While the array patches are simple and easy to use in the clinic, they are very sophisticated, only manufacturable using the most modern equipment and it is likely they will be very expensive to develop for mass vaccination.
About 800 million humans are injected with vaccines every year”
Most vaccines in use today are relatively cheap to make and are injected into patients with mass‑produced and inexpensive (five-cent) disposable syringes. About 800 million humans – equivalent to 10 percent of the world’s population – are injected with vaccines every year.23 In spite of the cost and difficulties of the cold chain these injections have been saving 3.5-5 million lives annually for decades.24
However, this enormously successful public health measure is recognised by the WHO as still falling short today.25 In 2021 an estimated 25 million children under the age of one year did not receive basic vaccines, and the number of completely unvaccinated children increased by five million since 2019. In addition to developing new vaccines for new diseases, there is need to develop more stable vaccines to overcome difficulties with access to vaccines… which are required to achieve the WHO’s strategic objective #1: “to reduce the health, social and economic burden of communicable diseases”.26
Vision for vaccine development
We need a class of new vaccines that are cost-effective to manufacture, easy to stockpile, easy to distribute to remote regions and easy to safely administer “
In summary, we need a class of new vaccines that are cost-effective to manufacture, easy to stockpile, easy to distribute to remote regions and easy to safely administer to children. Simple processes already exists that can enable the existing portfolio of refrigerated vaccines to be converted to room-temperature products. One example of such a technology is that recently used to convert a commercial tetanus-diphtheria vaccine usually requiring refrigeration at 2-8°C into a room temperature product that is stable and fully‑protective in Guinea pigs after storage at 45°C for 7-12 months.27
The beauty of this approach is that it does not require the addition of exotic materials, nor the development of new devices, nor the invention of demanding new manufacturing technologies. It has potential to be applied simply and directly to most existing commercial vaccines. Upon advice from the European Medicines Agency (EMA) confirming that toxicology is not required, and since the UK’s Medicines and Healthcare products Regulatory Agency (MHRA) has confirmed that a bioequivalence trial is sufficient for clinical trials approval, Stablepharma will be initiating GMP manufacturing in 2023 with a product launch anticipated in 2025. Since vaccines can now be reformulated to meet the relevant WHO guidelines, the route to a future of fridge-free vaccines is open.
About the author
Dr Bruce Roser MB BS, PhD founded Stablepharma in 2012, to bring to market his important work on vaccine stabilisation. Over his career as a physician and scientist he published 108 papers and 49 patents. He is a recognised world authority in drug stabilisation and in 1985 he patented the remarkable stabilising activity of trehalose glasses on vaccines and other biologicals.
References
- What percentage of the world population lives in developing countries? [Internet] Burak. 2022. [cited 2023July]. Available from: https://mywebstats.org/what-percentage-of-the-world-population-lives-in-developing-countries.
- Falcón VC, Porras YVV, Altamirano CMG., Kartoglu U. A vaccine cold chain temperature monitoring study in the United Mexican States. Vaccine. 2020;38:5202–5211.
- Matthias DM, Robertson J, Garrison MM, et al. Freezing temperatures in the vaccine cold chain: A systematic literature review. Vaccine. 2007;25:3980–3986.
- Kristensen D, Chen D, Cummings R. Vaccine stabilization: Research, commercialization, and potential impact. Vaccine. 2011;29:7122–7124.
- Sharma D, Khamar S, Cullen A, et al. Innovative Drying Technologies for Biopharmaceuticals. Int J Pharm. 2021;609.
- US10821210B2 Injections. [Internet] Roser BJ. 2013. [cited 2023July]. Available from: https://worldwide.espacenet.com/patent/search/family/045876206/publication/US10821210B2? q=US10821210B2.
- Heatstable vaccines urgently needed to reach the one in five children missed by immunisation worldwide. [Internet] Médecins Sans Frontières. 2014. [cited 2023July]. Available from: https://www.msf.org/heat-stable-vaccines-urgently-needed- reach-one-five-children-missed-immunisation-worldwide.
- Lee B, Wedlock PT, Haidari LA, et al. Economic impact of thermostable vaccines. Vaccine. 2017;35:3135-3142.
- Gribbon EM, Sen SD, Roser BJ, Kampinga J. Stabilisation of vaccines using trehalose (QT4) technology PMID: 8854017. Dev Biol Stand 1996;87:193–9.
- US5149653A Preservation Of Viruses. [Internet] Roser BJ. 1992 [cited 2023July]. Available from: https://worldwide.espacenet.com/patent/search/family/010630314/publication/US5149653A?q=US5149653.
- Roser BJ. Stable Liquid Drugs and Vaccines for the 21st Century. Future Microbiol. 2006;1:21–31.
- Green JL, Angel CA. Phase relations and vitrification in saccharide water solutions and the trehalose anomaly. J. Phys. Chem. 1989;93(8):2880–2.
- Roe KD, Labuza TP. Glass Transition and Crystallization of Amorphous Trehalose-sucrose Mixtures, Int. J. Food Prop. 2005;8(3): 559-574.
- Wolfenden R, Yuan Y. Rates of Spontaneous Cleavage of Glucose, Fructose, Sucrose, and Trehalose in Water, and the Catalytic Proficiencies of Invertase and Trehalase. J. Am. Chem. Soc. 2008;130 (24):7548–7549.
- Kumar V, Banker GS. Maillard Reaction and Drug Stability. I Woodhead Publishing Series in Food Science, Technology and Nutrition, Maillard Reactions in Chemistry, Food and Health. Woodhead Publishing. 2005. Pages 20-27. ISBN 9781855737921.
- Higashiyama T. Novel functions and applications of trehalose. Pure Appl. Chem. 2002;74 (7):1263–1269.
- TRS 953 – Annex 2, Appendix 1: Stability testing of active pharmaceutical ingredients and finished pharmaceutical products: Stability conditions for WHO Member States by Region. [Internet] WHO. 2021. [cited 2023July]. Available from: https://www.who.int/publications/m/item/trs953-annex2-appendix1.
- Alcock MG, Cottingham CS, Rollier J, et al. Long-term thermostabilization of live poxviral and adenoviral vaccine vectors at supraphysiological temperatures in carbohydrate glass. Sci Transl Med. 2010;2(19):ra12.
- Li AB, Kluge JA, Guziewicz NA, et al. Silk-based stabilization of biomacromolecules. J Control Release. 2015;10(219):416-430.
- Wahid AA, Doekhie A, Sartbaeva A, van den elsen JMH. Ensilication improves the thermal stability of the tuberculosis Antigen Ag85b and an Sbi-Ag85b Vaccine conjugate. Nature Scientific Reports. 2019; 9:11409.
- Li J, Zeng M, Shan H, Tong C. Microneedle Patches as Drug and Vaccine Delivery Platform. Curr Med Chem. 2017;24.
- vander Straeten A, Sarmadi M, Daristotle JL, et al. A microneedle vaccine printer for thermostable COVID-19 mRNA vaccines. Nat Biotechnol. 2023.
- Injection Safety Fact Sheet. [Internet] WHO. 2016. [cited 2023July]. Available from: https://cdn.who.int/media/docs/default-source/integrated-health-services-(ihs)/injection-safety/is_fact-sheet.pdf?sfvrsn=27f55c57_5.
- Vaccines and Immunization. [Internet] WHO. 2020. [cited 2023July]. Available from: https://www.who.int/health-topics/vaccines-and-immunization#tab=tab_1.
- WHO. Immunization coverage. [Internet] WHO. 2023. [cited 2023July]. Available from: https://www.who.int/news-room/fact-sheets/detail/immunization-coverage.
- Managing the threat of communicable diseases [Internet]. [cited 2023July]. Available from: https://apps.who.int/iris/bitstream/handle/10665/349964/WHO-EURO-2011-4331-44094-62196-eng.pdf#:~:text=WHO%20Strategic%20Objective%201%3A%20%E2%80%9CTo%20reduce%20the%20health%2C.
- de la Torre Arrieta J, Briceño D, de Castro IG, Roser B. A Thermostable Tetanus/diphtheria (Td) vaccine in the StablevaX™ pre-filled delivery system. Vaccine. 2023;41(22):3413-3421.
Issue
Related topics
Related organisations
European Medicines Agency (EMA), Médecins Sans Frontières (MSF), Medicines and Healthcare products Regulatory Agency, Stablepharma, The World Health Organization (WHO)