Polyelectrolyte complexes as nanoparticulate drug delivery systems
Posted: 3 July 2015 |
Polyelectrolyte complexes at the nanoscale (polyelectrolyte complex nanoparticles; PECNs) can be harnessed as drug delivery systems for a range of low molecular weight drugs as well as peptides and proteins. The mild method of manufacture of PECNs results in the protection of the loaded bioactive and the choice of polyions forming the carrier makes PECNs very flexible in the design of a range of medical and pharmaceutical applications. This paper presents the key characteristics of PECNs and developments in the area.
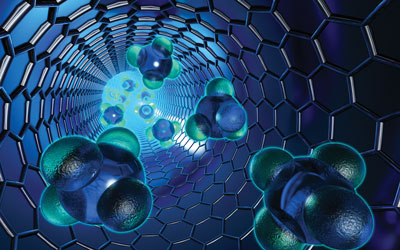
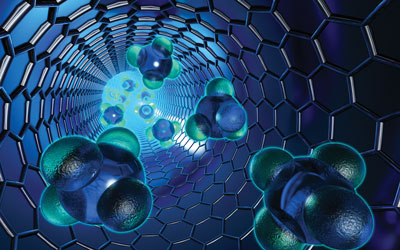
Nanoparticles (NPs) are currently being widely investigated for their medical applications as drug delivery systems as well as for diagnostic, medical imaging and tissue engineering purposes. Since nano technology offers a suitable means of controlled and/or site-specific delivery of low and high molecular weight drugs and macromolecules, considerable efforts have been dedicated to explore its potential use in drug delivery.1 The primary focus of pharmaceutical nanotechnology is formulation of active ingredients into biocompatible NPs. These systems offer many advantages over conventional delivery systems as they focus on efficacy of the active by improving its bioavailability and targeted delivery, improved safety, extending the molecule half-life in the target tissue and enhancing its stability against chemical and/or enzymatic degradation.
The submicron size is the key basis for many advantages of NP-based drug delivery systems due to enormous surface area of NPs and thus better absorption properties compared to larger carriers.1,2 It is very important for medical applications that NPs are biocompatible and biodegradable. Serious issues with respect to biocompatibility and toxicity are disadvantages of some NPs such as carbon nanotubes, fullerenes, quantum dots and other metal-based nanoparticles.
Components of PECNs – polymers and bioactives
There are many polymers at disposal for developing NPs for drug delivery. The following criteria determine whether a given polymer is deemed suitable for the delivery of drugs: biocompatibility and bioavailability (they have a crucial impact on disposition properties of PECNs), cytotoxicity and the degradation rate, which determines the rate of drug release and ease of production. The choice of materials also influences the size and morphology of the particles, the surface charge, drug loading and the desired release profile.3
Recently, there has been an increased interest in NPs composed of naturally occurring polymers such as polysaccharides or their derivatives due to their diverse structures, a large number of reactive groups, low toxicity, well established safety profiles, biodegradability and stability.4-10 Many of such molecules are polyelectrolytes – polymers with repeating units bearing either positive (polycations) or negative (polyions) charge. Indeed, those water-soluble, biodegradable polyelectrolytes degrade at a slow rate, do not alter normal cell functions and use water as a solvent in their fabrication, which is an important advantage for products that potentially may be applied as drug delivery systems in humans.
Such polymers are generally intended to be used solely as carriers for the delivery of bioactive substances, however some of them are known to possess pharmacological and functional properties as well. Examples of those polymers include chitosan (CS), hyaluronic acid (HA), chondroitin sulphate (CHON) and their application in nanomedicine could be multifold. In some cases the pharmacological properties of the polymers can be complementary to those of the encapsulated molecule. For instance, it has been shown that an intra-articular HA-calcitonin nanocomplex showed remarkable anti-inflammatory effects in vivo.10
To date the most appreciated natural polymer employed for NPs production is CS due to its mucoadhesive and permeation enhancer abilities and also thanks to the chemical properties (presence of cationic, charged groups), which allows the formation of NPs at relatively mild conditions of preparation.3 Chitosan NPs have been demonstrated to possess anti-bacterial properties and shown to improve the oral bioavailability of peptide and protein formulations as the positive charge of CS influences its reactivity with negatively charged surfaces, for example, cell and mucosal membranes.
There are many anionic polymers that can be complexed with CS to produce PECNs. Some of these are HA5,6,11, CHON, carboxymethylcellulose, dextran sulphate, alginate and polyaspartic acid4. HA is present in the components of the extra-cellular matrix of connective tissues and its action in vivo depends on the polymer size. Large (400-20,000 kDa) HA chains suppress angiogenesis and inhibit phagocytosis, while oligomeric hyaluronan fragments are angiogenic, immune-stimulatory and inflammatory.12 HA also interacts specifically with cell-surface receptors, e.g., CD44 and can act in synergy with CS to enhance mucoadhesion. Thus combining HA and CS into a nanoparticle may be a useful approach to form functional and targeted delivery systems. An example of such a system is that of HA/CS/calcitonin NPs, characterised by an efficient peptide association (above 90%), high drug loading (up to 50% w/w) with the loading capacity increasing with an increase in the HA content in NPs.6
CHON is an abundant glycosaminoglycan found in the cartilage, bone and connective mammalian tissue. It exhibits a wide variety of biological functions and is currently used as an anti-inflammatory, chondro-protective and anti-rheumatic drug. Similarly to HA, CHON has been shown to form a polyelectrolyte complex with CS.13 CHON/CS PECNs have been proven to be efficient carriers for the delivery of bovine serum albumin, growth factors and doxorubicin.
Other polycations used in the formation of PECNs are protamine7,9, polyarginine and polyethylenimine. Protamine (PROT) has been used in several marketed pharmaceutical products, e.g., insulin, for many years. It is utilised as an antidote for the neutralisation of an anticoagulant heparin. PROT also has cell-penetrating and nucleus-targeting properties and forms PECNs with different macromolecules, e.g., HA7, CHON8 and carrageenans9. HA/PROT NPs protected calcitonin against enzymatic degradation in vitro.7 The release of calcitonin from HA/PROT or CHON/PROT NPs depends on the type of polyanion as the peptide/NP complex is stronger in the CHON-based NPs compared with the HA-based NPs, therefore the former system provides a slower release of the peptide. Also, complexation to HA or CHON markedly reduces toxic effects exerted by PROT and the NPs are compatible and well-tolerated by Caco-2 cells.7,8 Table 1 presents short characteristics of selected polyelectrolytes that has been shown to successfully form PECNs.
Proteins have also been used in PECN-based drug delivery systems. For instance, gelatin has been used in the synthesis of NPs for drug delivery over the last thirty years. Gelatin is a natural, water soluble macromolecule obtained from the heat dissolution and partial hydrolysis of collagen. Also, albumins such as bovine serum albumin can be employed as either a nanocarriers for the delivery of bioactive molecules, e.g., siRNA, or as the active ingredient encapsulated into NPs. Human serum albumin is used as a protective colloid in albumin/PROT/oligonucleotide NPs, overcoming the disadvantages of PROT/oligonucleotide NPs, i.e., improving the stability and preventing aggregation under isotonic conditions.
Formation and formulation of PECNs
PECNs are usually obtained by simple and mild methods presenting the benefit of producing organic solvent-free and surfactant-free formulations. The main techniques employed are ionic gelation (cross-linking) or coacervation (phase separation) with the latter not requiring any extra excipients in addition to the polymers and the bioactive.
Coacervation caused by the interaction of two oppositely charged species is referred to as a complex coacervation (Figure 1). However, in many cases, the complexes are solid-like (precipitate) in nature. The PEC formation is mainly caused by strong Coulomb interactions between oppositely charged polyelectrolytes, however other inter-macromolecular interactions, e.g., hydrogen bonding, van deer Waals forces, hydrophobic interactions or dipole-charge transfer may also be involved in the formation of polyelectrolyte complexes.
The process of PECN formation should be conducted in diluted solutions to prevent macroscopic gelation leading to aggregation and hydrogels. Some of the factors determining the formation and properties of polyelectrolyte complexes are the strength and location of ionic sites, polymer chain rigidity, pH, temperature, ionic strength and mixing intensity.14 However, a coacervation process is normally carried out in mild conditions, without the use of organic solvents and/or surfactants, at room temperature and no special equipment is required.
In relation to formulation, the key parameter determining the success of formation and physical/colloidal stability of PECNs is the polyelectrolyte charge mixing.5-8 Since the colloidal stability of PECNs depends on the repulsion between similarly charged NPs, when the charge is neutralised, instability and aggregation occurs. Therefore the net charge of the system must be either strongly positive or strongly negative to be physically stable. Polyelectrolyte complexes are known to be unstable when the polycation and polyanion charge mixing ratio is close to 1.11 Other factors influencing the properties of PECNs are the type of polymers used (molecular weight, charge density, counterion and deacetylation degree for chitosan), polymer concentration as well as the composition, pH and ionic strength of the medium.5-9
Properties of PECNs relevant for drug delivery
The critical determinant of the fate of orally administered NPs is the particle size and size distribution, since particle transport through the intestinal epithelium is size-dependant.2,3 The particle size is mainly influenced by the polymer’s molecular weight. The extent of NP uptake is also governed by their surface properties: charge and hydrophobicity, influenced by the nature of polymer(s) and their composition as well as the presence of coating and attached targeting moieties. The surface charge of PECNs can easily be manipulated by changing the mass mixing ratio of the oppositely charged polymers.5-9 Figure 2 shows, based on selected PECNs examples, the impact of the polyanion:polycation mass mixing ratio on the size (diameter) and zeta potential of forming particles.
A successful nanocarrier is one which is characterised by a high loading capacity, as this would reduce the dose of NPs required for administration.15 Generally, the active ingredient may be loaded into the particles either by incorporation of the drug at the time of production of the NPs or by adsorption of the drug after NP formation by incubating NPs in the drug solution. While encapsulating a bioactive, one has to keep in mind its activity after the process, i.e., integrity of the compound. It is one of the most important parameters influencing the bioavailability and pharmacological efficacy of the active.3 As many biomolecules, e.g. proteins, are heat and/or solvent sensitive, mild and aqueous based-manufacturing processes (such as those used in the PECNs formation) are ideal for keeping the bioactive intact.
The release profile of the bioactive is affected by the character of polymers comprising the formulation. Polymers from natural sources usually provide a relatively quick release of the drug, while synthetic polymers are characterised by a more sustained release of the active ranging from days up to weeks, even months.3 The nature (e.g., whether hydrophilic or hydrophobic properties) and other physicochemical characteristics will determine and significantly impact on the mechanism of drug release and the overall release profile. The release of the active molecule and the properties of PECNs are dependent on the composition and ionic strength of the medium.8 The principal advantages and disadvantages of PECNs as drug delivery systems are summarised in Table 2.
Future of PECNs
In summary, the current research efforts in the area of pharmaceutical nanotechnology, especially polyelectrolyte complex NPs, encourage further developments of such systems. Given the complex interplay between the properties of the carrier components and the bioactive(s) as well as the formulation process, caution must be exercised and all critical material quality attributes and process parameters explored to ensure optimum PECNs for the application. The future of PECNs, however, is optimistic considering the range of benefits they offer.
References
- Hamidi M, Azadi A, Rafiei P. Hydrogel nanoparticles in drug delivery. Adv Drug Deliv Rev. 2008; 60: 1638-1649
- Alonso MJ. Nanomedicines for overcoming biological barriers. Biomed Pharmacother. 2004; 58: 168-172
- des Rieux A, Fievez V, Garinot M, Schneider Y-J, Préat V. Nanoparticles as potential oral delivery systems of proteins and vaccines: a mechanistic approach. J Control Release. 2006; 116: 1-27
- Liu Z, Jiao Y, Wang Y, Zhou C, Zhang Z. Polysaccharides-based nanoparticles as drug delivery systems. Adv Drug Deliv Rev. 2008; 60: 1650-1662
- Umerska A, Paluch KJ, Inkielewicz-Stepniak I, Santos-Martinez MJ, Corrigan OI, Medina C, Tajber L. Exploring the assembly process and properties of novel crosslinker-free hyaluronate-based polyelectrolyte complex nanocarriers. Int J Pharm. 2012; 436: 75-87
- Umerska A, Corrigan OI, Tajber L. Intermolecular interactions between salmon calcitonin, hyaluronate, and chitosan and their impact on the process of formation and properties of peptide-loaded nanoparticles. Int J Pharm. 2014; 477: 102-112
- Umerska A, Paluch KJ, Martinez MJ, Corrigan OI, Medina C, Tajber L. Self-assembled hyaluronate/protamine polyelectrolyte nanoplexes: synthesis, stability, biocompatibility and potential use as peptide carriers. J Biomed Nanotechnol. 2014; 10: 3658-3673
- Umerska A, Paluch KJ, Santos-Martinez MJ, Medina C, Corrigan OI, Tajber L. Chondroitin-based nanoplexes as peptide delivery systems – Investigations into the self-assembly process, solid-state and extended release characteristics. Eur J Pharm Biopharm. 2015; 93: 242-253
- Dul M, Paluch KJ, Kelly H, Healy AM, Sasse A, Tajber L. Self-assembled carrageenan/protamine polyelectrolyte nanoplexes-Investigation of critical parameters governing their formation and characteristics. Carbohydr Polym. 2015; 123: 339-349
- Ryan SM, McMorrow J, Umerska A, Patel HB, Kornerup KN, Tajber L, Murphy EP, Perretti M, Corrigan OI, Brayden DJ. An intra-articular salmon calcitonin-based nanocomplex reduces experimental inflammatory arthritis. J Control Release. 2013; 167: 120-129
- Boddohi S, Moore N, Johnson P, Kipper M. Polysaccharide-based polyelectrolyte complex nanoparticles from chitosan, heparin and hyaluronan. Biomacromolecules. 2009; 10: 1402-1409
- Stern R, Asari AA, Sugahara KN. Hyaluronan fragments: an information reach system. Eur J Cell Biol. 2006; 85: 699-715
- Denuziere A, Ferrier D, Domard A. Chitosan- chondroitin sulfate and chitosan-hyaluronate polyelectrolyte complexes. Physico-chemical aspects. Carbohydr Polym. 1996; 29: 317-323
- Hartig SM, Greene RR, Dikov MM, Prokop A. Davidson JM. Multifunctional nanoparticulate polyelectrolyte complexes. Pharm Res. 2007; 24: 2353-2369
- Soppimath KS, Aminabhavi TM, Kulkarni AR, Rudzinski WE. Biodegradable polymeric nanoparticles as drug delivery devices. J Control Release. 2001; 70: 1-20
Biographies
Dr. Anita Umerska studied Pharmacy at Medical University of Gdansk, Poland. She worked as a pharmacist in the UK for two years. She received her PhD from Trinity College Dublin, Ireland in 2013. Currently she is a post-doctoral researcher at University of Angers, France. She is interested in engineering of nanocarriers for a variety of applications.
Dr. Lidia Taber obtained her MPharm degree from the Medical University of Silesia, Poland in 2000. She then joined Trinity College Dublin (TCD) to pursue her PhD and currently is employed as Assistant Professor in Pharmaceutical and Pharmaceutical Sciences in TCD. Her research interests include solid state pharmaceuticals and pharmaceutical nanotechnology.