Cancer biology where do we go next?
Posted: 22 February 2010 | Dr Anthony Davies, Director of High Content Research Facility, Department of Clinical Medicine; Dr Anthony McElligott, Research Scientist, Institute of Molecular Medicine; Dr Graham Pidgeon, PhD., Senior Lecturer in Surgery/Molecular Oncology, Institute of Molecular Medicine; Dr Anthony Ryan, Research Scientist, Institute of Molecular Medicine, Trinity College Dublin and Dr Ruth Foley, Prostate Cancer Research Group
The World Cancer Report (2008) predicts a 50% worldwide increase in cancer incidence by 2030, predicting 75 million people living within a five year diagnosis of cancer1. This increase is partially fuelled by significant medical advances in developed countries ensuring people live longer. However, it is also attributable to developing countries adopting habits linked to cancer risk such as increased uptake of smoking and the acquisition of western diets. In 2007, cancer caused approximately 7.6 million or 13% of all human deaths2. Cancers associated with the greatest mortalities are lung, stomach, colorectal, liver and breast cancer respectively. There are modifiable risk factors common to many malignancies, including tobacco, overweight or obesity, poor physical activity, dietary factors, alcohol, sunlight exposure and chronic infection. Effective prevention will reduce the risk of cancer, and efficient screening will enable many to be successfully treated for their disease.
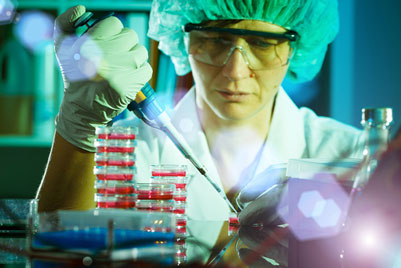
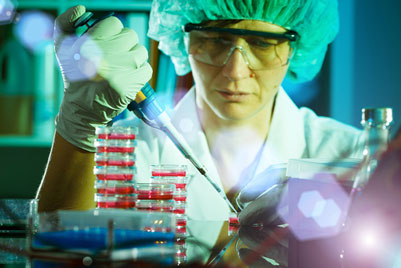
The World Cancer Report (2008) predicts a 50% worldwide increase in cancer incidence by 2030, predicting 75 million people living within a five year diagnosis of cancer1. This increase is partially fuelled by significant medical advances in developed countries ensuring people live longer. However, it is also attributable to developing countries adopting habits linked to cancer risk such as increased uptake of smoking and the acquisition of western diets. In 2007, cancer caused approximately 7.6 million or 13% of all human deaths2. Cancers associated with the greatest mortalities are lung, stomach, colorectal, liver and breast cancer respectively. There are modifiable risk factors common to many malignancies, including tobacco, overweight or obesity, poor physical activity, dietary factors, alcohol, sunlight exposure and chronic infection. Effective prevention will reduce the risk of cancer, and efficient screening will enable many to be successfully treated for their disease.
In 1957, carcinogenesis was first recognised to be a multistage process, with each stage determined by the activation, mutation or loss of specific genes3. In the majority of cases, cancer arises from a single cell which has acquired some heritable growth advantage. This initiation step is believed to be frequently caused by radiation or chemical carcinogens. Further mutational events affecting the parameters of cell growth can result in the emergence of clones of cells with specific properties associated with tumour cell progression. Metastatic ability is achieved within these clones, following final gene changes and/or alterations. Numerous animal models of carcinogenesis, primarily developed in mice, have enabled the broader classification of these steps into initiation events, promotion, transformation and metastasis4.
In order for a normal cell to transform into a cancer cell, the genes which regulate cell growth and differentiation must be altered5. Genetic changes can occur at many levels, from gain or loss of entire chromosomes, to mutations affecting a single DNA nucleotide6. Over the past 30 years, considerable information has been gathered on the genetic regulation of cell growth leading to the identification of two broad categories of genes influencing cancer growth, proto-oncogenes and tumour suppressor genes7. Proto-oncogenes encode proteins that are predominantly involved in cell proliferation, differentiation, cell cycle control and apoptosis. Tumour suppressor genes are genes which inhibit cell division, survival, or other properties of cancer cells and are often disabled by cancer-promoting genetic changes.
Typically, changes in multiple genes are required to transform a normal cell into a cancer cell. Deregulation in the fine balances controlling cellular proliferation and cell death are major hallmarks of cancer8. During normal cell growth, there is a finely controlled balance between growth-promoting and limiting signals to ensure proliferation occurs only when required. This balance is titled when increased cell numbers are required, for example during wound healing or during normal tissue turnover. The differentiation of cells during these processes occurs in an orderly manner. In tumour cells, this process is disrupted resulting in continued cell proliferation, and in many cases loss of differentiation.
In the foreseeable future, it is unlikely that a single approach will be used to prevent or treat cancer until the exact mechanisms responsible for tumour development have been unravelled. Translational research is critical if we are to delineate these mechanisms, enabling the movement of scientific discovery into new approaches for cancer treatment, or translating information about cancer risk factors into changes in population behaviour. Within the field of molecular biology, imaging will enable clinicians to not only localise tumours in the body, but also to visualise the expression and activity of specific molecules and biological processes (e.g., apoptosis, angiogenesis, and metastasis) that ultimately influence tumour behaviour and response to therapy. This information is expected to influence cancer screening, individualised treatment, and drug development, in addition to increasing our understanding of how cancer arises.
Challenges in cancer research
In the last hundred years, over two million papers have been published on cancer, and vast sums of money have been invested in cancer research. Despite the many significant advances that have been gained though these extensive research programs and the colossal effort and investment that is still ongoing, the question is why are patients still dying of cancer?
A key issue here is that cancer is not only a complex disease, but a diverse one. For example two patients may receive the same treatment for tumours of identical type and stage, but only one may respond and survive. Clearly there are uncharacterised but important differences in how the tumour cells in these patients respond to treatment. Many studies have identified molecular signatures characteristic of a clinically-defined subset of tumours from one tissue, but as a consensus rather than as a uniform set of molecular features. These layers of variability, between tissues, disease stages and patients, mean that insights into a specific subset of tumours cannot be assumed to apply to all other types of malignancy, and that considerable resources are required to properly understand the disease.
Challenges in treating cancer
A major difficulty in treating cancer is achieving a balance between successfully killing all cancer cells and avoiding severe side-effects. Normal cells have the capacity to divide, differentiate or undergo apoptosis, and precise regulation of these processes allows tissues to maintain homeostasis. Inappropriate cell division, and failure of cells to undergo apoptosis, are key underlying factors in cancer development. Mutagenic radiation and chemotherapeutic drugs aim to target cancer cells on the basis that they are dividing. However, these strategies do not always distinguish adequately between cancer cells and dividing benign “normal cells”.
A further challenge is posed by inherent and acquired resistance to cancer treatment. The alterations that make cells malignant often contribute to their resistance to current cancer therapies. Cancer cells with p53 mutations can bypass cell cycle checkpoints, which would normally cause cells to undergo apoptosis when DNA is damaged. Such changes give cancer cells a selective advantage over normal cells under adverse conditions. While imatinib mesylate has revolutionised the treatment of chronic myeloid leukaemia, secondary or acquired resistance to the drug is a significant issue. Treatment selects for leukaemia cells with mutations which reduce or eliminate drug binding to the target protein bcr-abl, and second-line bcr-abl inhibitors are in development9. Also, hypoxia is a feature of many solid tumours, due to the disorganised tumour blood vessel network, and cellular adaptation to hypoxic stress increases resistance to treatment.
Early detection is the key
The final challenge to consider here is that of detecting cancer early enough to treat it effectively. Detection at an early grade and stage is usually a crucial factor influencing treatment success and survival. Overall, metastatic disease accounts for 90% of cancer-related deaths. Clearly, early detection will always be easier to achieve for some tissues than others. Most newly diagnosed melanoma skin cancer cases are localised, with an excellent five year survival rate of over 95%10. At the other extreme, the majority of ovarian cancers are metastatic at diagnosis, as they do not cause symptoms at earlier stages.
The challenges of cancer research and treatment thus include molecular complexity, disease diversity, treatment-related toxicity, treatment resistance and early diagnosis. However, through innovative and strategic research programmes, cancer care will continue to overcome these.
As with any biological phenotype, genetics also plays a role in cancer development
Genetic variants such as the BRCA genes (involved in genome stability) which were identified in the 1990s as leading to familial forms of breast cancer. These are single gene (or Mendelian) forms of inheritance, where genetic variability at a single locus leads to the disease. However, even with single gene disorders, the penetrance (the probability of getting the disease, given the presence of the genotype) can vary considerably. The OMIM database (Online Mendelian Inheritance in Man, http://www.ncbi.nlm.nih.gov/sites/OMIM) catalogues over two thousand cancer related genes. Mendelian inheritance of a disease often involves rare variants of individual genes, which are identified in large pedigree studies, where the genes and the inheritance of disease are followed simultaneously.
However, many common forms of disease, and cancer is no exception, clearly do not exhibit Mendelian inheritance. These are referred to as complex (or polygenic) diseases, in which the development of disease depends on the interaction of many genetic and environmental factors. In complex disease, each individual genotype can be viewed as a risk factor. If an individual carries enough of these risk factors, and has the right environmental exposure, the chances of disease are increased.
Numerous large-scale studies, for example those of the Wellcome Trust Case-Control Consortium (http://www.wtccc.org.uk/), have investigated the association between single nucleotide polymorphisms (SNPs) and numerous disease phenotypes, on a genome wide scale. This includes analysis of breast cancer susceptibility for non-synonymous coding SNPs. The International Lung Cancer Consortium (http://ilcco.iarc.fr/) has focussed on SNPs in lung cancer susceptibility, in addition to non-genetic risk factors.
The known functional impact of SNPs includes amino acid substitution, changes in gene expression patterns and alteration of splicing patterns. In addition, recent advances in the understanding of RNA interference have the potential to contribute both to the understanding of the aetiology of specific diseases as well as the investigation of gene function by siRNA knockdown.
Genetic approaches not only identify the genetic variants which predispose to disease, but also to shed light on the relevant pathways in the aetiology, which can in turn inform later functional studies, including cell-based assays and cell imaging and analysis.
Where does cellular based imaging and analysis fit in?
Cell based assays offer new insights
As can be seen, it is clear that one single approach to the study and/or the detection of cancer will not provide us with the detailed information we require, to develop a sufficient understanding of the mechanisms that underlie the development and progression of cancer. However the appropriate use of cell based assays with disease relevant cell lines or primary cells, do offer the capability of unravelling the complexity of molecular interactions at the cellular level. Indeed to improve physiological relevance even further many researchers are now turning to 3 dimensional cell culture systems. Many of those who have conducted studies with 3 dimensional cell based systems have reported demonstrable differences between cancer cells grown in 3D Vs 2D culture. These differences seem to be reflected in the cells ability to resist the effects of anti cancer drugs. Reports from various sources indicate that cell grown in 3D culture exhibit an elevated or enhanced chemo-resistance compared with the same cells grown 2D conditions. Ultimately this new approach offers the capability of developing new and improved cellular models for the study of drug resistance in cancer. In conjunction with these new types of methodological approaches, the technology surrounding this scientific area i.e. image acquisition and analysis technologies now currently make these types of studies possible in a screenable format.
Cellular imaging and analysis technologies
In a broader context Cellular imaging and analysis technologies (such as HCA) with medium throughput capabilities have promise in generating phenotypic profiling in response to small molecule screening. These technologies provide single cell resolution and make quantitative measurements of cellular parameters using fluorescent marker intensity and morphological measurements. A major challenge of this technology is the ability to mine and integrate the large data sets and extract meaningful biological data from images produced by these systems. Despite these challenges, the integration of this high-content analysis of phenotypic cellular changes with target identification has proven to be successful in increasing the selection of lead compounds for the cancer drug discovery process, offering hope of new treatments in the foreseeable future.
References
- WHO (2008). “World Cancer Report 2008”. World Health Organization. http://www.iarc.fr/en/publications/pdfs-online/wcr/2008/wcr_2008.pdf.
- American Cancer Society (December 2007). “Report sees 7.6 million global 2007 cancer deaths”. Reuters. http://www.reuters.com/article/health News/idUSN1633064920071217. Retrieved 2008-08-07.
- Foulds L (1957). Cancer Research 17:355.
- Nowell P.C. (1976). Science 194: 23.
- Croce CM (January 2008). “Oncogenes and cancer”. The New England journal of medicine 358 (5): 502-11.
- Rabbitts TH. Chromosomal translocations in human cancer. Nature. 1994 Nov 10; 372 (6502):143-9.
- Greenberg AH, Egan SE, Wright JA. (1989) Oncogenes and metastatic progression. Invasion Metastasis. 1989; 9(6):360-78.
- Hanahan D, Weinberg RA (2000).The hallmarks of cancer. Cell. 2000 Jan 7;100(1):57-70.
- Tanaka, R. and S. Kimura, Abl tyrosine kinase inhibitors for overriding Bcr-Abl/T315I: from the second to third generation. Expert Rev Anticancer Ther, 2008. 8(9): p. 1387-98.
- Balch, C.M., et al., Final version of 2009 AJCC melanoma staging and classification. J Clin Oncol, 2009. 27(36): p. 6199-206.
Further reading
Kuchenbauer, F., et al., In-depth characterization of the microRNA transcriptome in a leukemia progression model. Genome Res, 2008. 18(11): p. 1787-97.
Kim, E.K. and E.J. Choi, Pathological roles of MAPK signaling pathways in human diseases. Biochim Biophys Acta.
About the author
Anthony Davies
Dr Davies received his PhD in molecular Cardiology from the Faculty of Medicine, University of Dundee Scotland. Following the completion of this research project he joined Tayside Institute of Child Health, Dundee, Scotland where he focused on the development of specialized cell based assays and perfusion systems for the study of hypoxic and ischemic insult in cardiac muscle cells and tissues. Dr Davies undertook further research in the IBLS (University of Glasgow) where he focused on the development of cell based assays and living cellular arrays for use with high throughput and high content screening of cardiac cells. Dr Davies now holds a senior position as Director of high content research facility (Trinity HCA) Department of Clinical Medicine Trinity College Dublin.