At the crossroads: The next decade
Posted: 26 April 2012 |
Flow cytometry is the technology that has the most impact on single-cell analysis. Over the past 40 years, it has arguably been the single most important research technique in the fields of basic and applied immunology. Flow cytometry excels in quantitative evaluation of receptor expression, separation of functionally defined cell populations and monitoring of cellular differentiation. For the clinical sciences, flow cytometry has been a key tool for diagnostics whereby aberrant populations are identified, classified and quantified, and in situations such as minimal residual disease, is capable of identifying rare cells indicative of dysplasia.
Despite the importance of its applications, flow cytometry is often seen as an aging technique without new exciting opportunities. The reality could not be further from the truth. The systems biology approach breathes new life into this unique technology. The new challenges of complex analysis of networks and pathways are a natural fit for modern multifactorial flow cytometry.
At the dawn of modern immunology, Len Herzenberg at Stanford University demonstrated the power of this high-tech tool in the early 1970s and it was soon the select tool for immunologists1. The advantage of flow cytometry was clear to most.
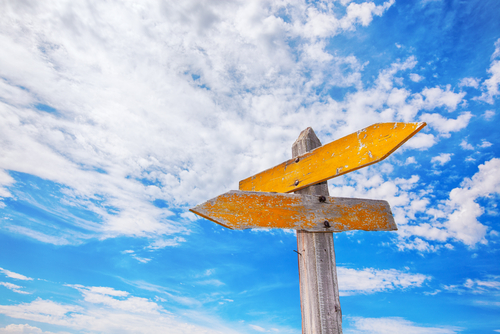
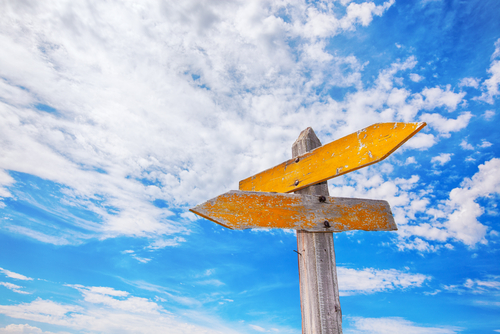
Flow cytometry is the technology that has the most impact on single-cell analysis. Over the past 40 years, it has arguably been the single most important research technique in the fields of basic and applied immunology. Flow cytometry excels in quantitative evaluation of receptor expression, separation of functionally defined cell populations and monitoring of cellular differentiation. For the clinical sciences, flow cytometry has been a key tool for diagnostics whereby aberrant populations are identified, classified and quantified, and in situations such as minimal residual disease, is capable of identifying rare cells indicative of dysplasia.
Despite the importance of its applications, flow cytometry is often seen as an aging technique without new exciting opportunities. The reality could not be further from the truth. The systems biology approach breathes new life into this unique technology. The new challenges of complex analysis of networks and pathways are a natural fit for modern multifactorial flow cytometry.
Introduction
At the dawn of modern immunology, Len Herzenberg at Stanford University demonstrated the power of this high-tech tool in the early 1970s and it was soon the select tool for immunologists1. The advantage of flow cytometry was clear to most. It could analyse multiple populations of cells simultaneously, so it was perfect for work with blood and bone marrow. Over the past few decades, the number of fluorescent markers that could be simultaneously measured from single cells in flow cytometry increased from just a few to 172. Since the combination of markers defines the cell function, the number of available populations grew from n2 to n17, where n is the number of recognisable intensity expression levels. Since the defined populations come from a complex biological system, one may search for underlying, biologically meaningful relationships between them. For a simple experiment involving just eight fluorescent markers describing cells as ‘positive’ or ‘negative’ for the presence of any given marker, up to 256 potential populations can be defined. This leads to 32,640 pairwise relationships between the observed populations. Perhaps those in the field of flow cytometry are just too conservative. Until recently, the focus of most studies using multiparametric flow cytometry was to identify small yet important populations of cells rather than to investigate complex relationships between populations in the system. This is where the field is going through a metamorphosis; it has begun its move into the world of systems biology.
Flow cytometry of the past
Traditionally, flow cytometry data analysis uses simple 1-D and 2-D visualisation to identify and separate populations of cells on the basis of measured signal intensities and other optical features, such as scattered light. The fluorescence signals coming from labels bring information about functional properties of cells, such as expression of cell surface markers, while light scatter can be linked to size, granularity or a combination of these variables. Frequently, flow cytometry has been used to find a unique population of cells and then explore the functional properties of these cells. From here, the advantage of flow cytometry would appear to be related to its unique capacity to separate cells into populations. This has been the fundamental advantage of the technology, enhanced by the fact that it is the most exquisite single-cell technology available. The problem is that while flow cytometry has proven its worth in basic immunology, clinical analysis, microbial analysis and a variety of other fields, these core applications may have reached their natural limits of expansion.
The changing paradigm of high content
But what has changed in the last couple of years? Complexity. In flow cytometry, the complexity in studies of biological samples can not only be accessed and analysed owing to the huge number of simultaneous functional measurements, but also via multifactorial experiment design, in which a biological system under analysis is exposed to multiple combinations of conditions (such as presence of activators, drugs in different concentrations, other biological or chemical perturbants, different temperature or time point post activation, etc.). Consequently, given the fact that the sample of every population is interrogated in multiple states, the number of potential relationships increases even further. The action of some perturbations is known as a priori (or can be predicted from a model). The process of integrating information about cellular responses provided by flow cytometry with biological models brings high-throughput flow cytometry into the domain of systems biology3. For example, recent work by Nolan has shown that high-content flow cytometry is fully capable of defining complex signalling pathways either directly or indirectly4-6. Next generation flow cytometry will become a tool that describes and defines an entire biological system rather than any small component, such as a selected population of cells.
Next-generation tools – networks and pathway analysis
So what will the next generation of flow cytometry be doing? Will it be defining the percentages of T cells and their many subsets or determining the number of receptors expressed on a subset of cells? There is no doubt that the current applications employing traditional flow cytometers will remain crucial for basic research and clinical studies. However, these will not provide further motivational impulse to improve flow cytometry technology. On the contrary, the quickly growing fields such as systems biology will allow flow cytometry to expand its application and become an effective tool for studying how drugs modify cell metabolism or stimulate protein phosphorylation, increase or decrease an enzyme’s abundance, or push a cell through a differentiation pathway.
How is this is going to happen? The first requirement for next-generation FC is that it has an ability to investigate significantly more complex biological questions and define a process for reducing an investigation to an experimental protocol. Four components must be met: the biological problem must be clearly stated, the reagents / labels must be available, the detection / measurement systems must be established and finally, a data-processing toolkit must be available to reduce the complex measurements to an understandable result. The best example of this is the work coming from Nolan’s laboratory, which is focused on understanding the signalling pathways and networks involved in driving chronic myelogenous leukaemia. The key area to be understood in this case is the impact of drug activity on multiple cellular populations isolated from bone marrow. Because there are many cellular populations present (14 or more) and the source of the biological sample is limited (patient bone marrow), the only way to ascertain the nature of activation pathways is to create a highly multiplexed assay system that evaluates all cell populations with all possible pathway markers with all possible activators simul – taneously. Although such experiments are difficult to plan, execute and interpret, these studies represent the opening gambit for the future of multifactorial cytometry (ref: Bodenmiller in press).
The systems biology opportunity
Systems biology requires large scale tools that usually generate dense data sets focused on a broad question.The systems approach requires integration of large multifactorial datasets so that a model of interactions between the various components can be derived (the sum is bigger than its parts) which in turn can then be validated using focused experiments. For the greater part, the systems biology domain has been driven by genomic and proteomic technologies using gene or protein sequencing, microarrays, and highthroughput screening technology.
However, the great majority of these assays have a very large number of measured param – eters, often in multifactorial format. The measurements are not linked to a particular well-defined cell phenotype, because so far these technologies have relied on the analysis of heterogeneous cell samples rather than narrowly selected cellular populations. Here VHC flow cytometry, which can easily separate populations defined on the basis of function / phenotype, has a tremendous advantage and is already showing significant impact. A recent development, mass spectro – metry–based flow cytometry (mass cytometry), has set out to match current ‘omic’ technologies in terms of data amount generated. Instead of measuring fluorescence at multiple wave – lengths (‘colours’), mass cytometry analyses the quantity and mass of stable isotopes attached to cells by atomising single cells and measuring the resulting ions in an atomic mass spectrometer. This approach already allows the quantification of ~40 parameters on the single-cell level with a throughput and sensitivity similar to fluorescent cytometry. Given that many stable metal isotopes are available (~100)7, even higher dimensionality of measurements will become feasible. This opens exciting new avenues for systems biology analysis: not only the cellular phenotype, but also signalling and protein network state, mRNA levels, etc., can be measured comprehensively and simultaneously for every single cell in heterogeneous cell mixtures within cellular developmental continua. Combining this technique with drug or siRNA-screening approaches to study different diseases models will not only greatly deepen our understanding of the underlying biology, but might also point out new avenues for the development of treatments. Overall, there is no doubt that the complexity of modern flow cytometry has been extended well beyond that of many other technologies, creating opportunities for many new applications.
The future of flow cytometry?
Considered a ripe and mature research technique serving mostly traditional fields of study just a few years ago, flow cytometry has been impacted and dramatically rejuvenated by the systems biology approach. The introduction of new signal-acquisition techniques as well as integration with lab automation and robotics made it possible to collect multidimensional and multifactorial data on complex hetero – geneous cell populations and to analyse their underlying cellular networks. This is really transformational and creates many new and quite different alternatives for flow cytometry studies. While new technologies such as mass cytometry are going to have a strong impact, there is plenty of opportunity for exciting studies using more traditional fluorescence based flow cytometry. Mass cytometry is slow and takes a significant time to collect a large number of cells. Traditional cytometry is capable of tremendous throughput and can be matched with robotics as described above, or barcoded data sets which increase the multiplexing significantly. New instruments in the traditional flow cytometry field are faster, offer more standard colours (at least 10) and have multiple excitations. Many are autoaligning and provide very high quality, robust and reproducible data. Date analysis is going to be the primary obstacle to broad deployment. As the complexity of samples increases, there is a commensurate increase in difficulty in data analysis. It is complex enough with traditional flow cytometry but the generated large-scale data sets from mass cytometry are very complex, and the development of computational tools lags behind the biology. Advances in FC data processing are urgently needed to fully realise the potential of this revitalised and rapidly expanding technology.
References
1. Bonner, WA., Hulett, HR., Sweet, RG and Herzenberg, LA; Fluorescence activated cell sorting, Review of Scientific Instruments 43:404-409, 1972
2. Perfetto, SP., Chattopadhyay, PK and Roederer, M; Seventeen-colour flow cytometry: unravelling the immune system, Nat.Rev.Immunol. 4:648-655, 2004
3. Krutzik, PO., Bendall, SC., Hale, MB., Irish, JM and Nolan, GP; Phospho Flow Cytometry: Single Cell Signaling Networks in Next Generation Drug Discovery and Patient Stratification, Flow Cytometry in Drug Discovery and Development 303-334, 2011
4. Linderman, MD., Bruggner, R., Athalye, V., Meng, TH., Bani Asadi, N and Nolan, GP; High-throughput Bayesian network learning using heterogeneous multicore computers, 95-104, 2010
5. Qiu, P., Simonds, EF., Bendall, SC., Gibbs Jr, KD., Bruggner, RV., Linderman, MD., Sachs, K., Nolan, GP and Plevritis, SK; Extracting a cellular hierarchy from high-dimensional cytometry data with SPADE, Nature Biotechnology 2011
6. Sachs, K., Perez, O., Pe’er, D., Lauffenburger, DA, and Nolan, GP; Causal protein-signaling networks derived from multiparameter single-cell data, Science 308:523-529, 2005
7. Bendall, SC., Simonds, EF., Qiu, P., Amir, E., Krutzik, PO., Finck, R., Bruggner, RV., Melamed, R., Trejo, A., Ornatsky, OI., Balderas, RS., Plevritis, SK., Sachs, K., Pe’er, D., Tanner, SD and Nolan, GP; Single-cell mass cytometry of differential immune and drug responses across a human hematopoietic continuum, Science 332:687-696, 2011