Antibody-drug conjugates: challenges, solutions and future potential
Posted: 20 April 2021 | Dave Elder (David P Elder Constultancy), Stacey Treichler (Catalent) | No comments yet
The ability to precisely direct powerful therapeutics not only makes treatments more effective, but also prevents debilitating side effects. Here, Dave Elder and Stacey Treichler highlight the great potential of antibody-drug conjugates (ADCs) for improved treatment of oncology indications and beyond.
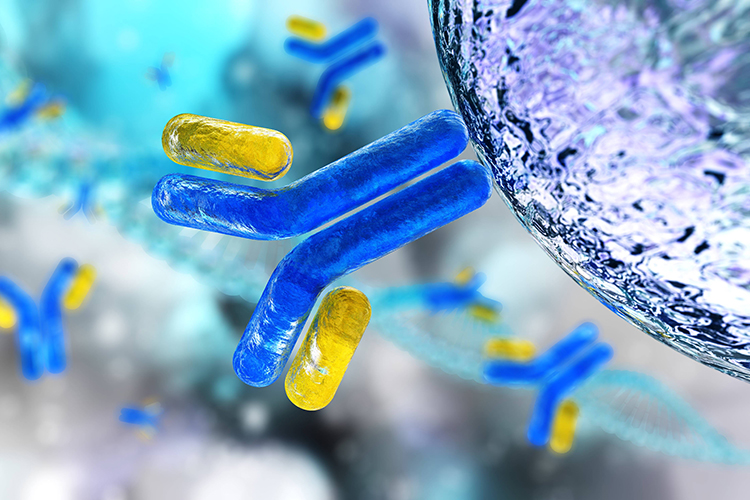
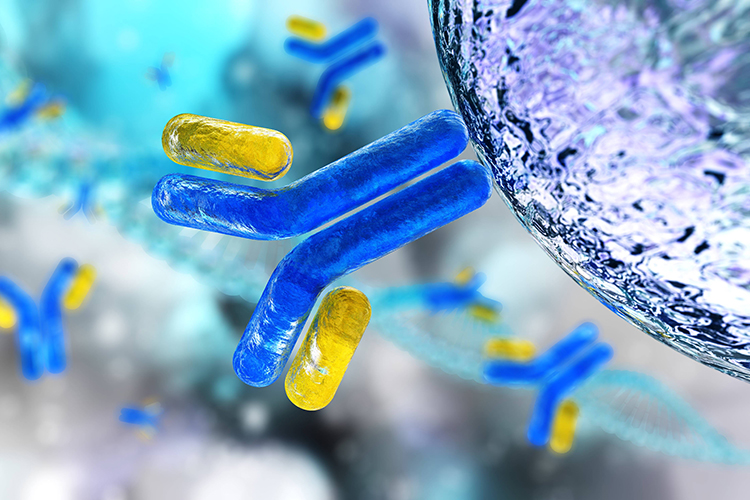
TARGETED DRUG DELIVERY is the ability to accurately target molecules to their site of action, minimising unwanted side effects. In conventional chemotherapy, cytotoxic drugs are delivered orally or systemically, but their efficacy is often compromised by extensive side effects. In contrast, antibody-drug conjugates (ADCs) can deliver potent anti-cancer agents – that are often too toxic to be dosed systemically on their own – directly to cancer cells, thereby minimising unwanted systemic toxicity. ADCs, a type of bioconjugate/immunoconjugate, work by chemically linking the potent anti-cancer agent to a monoclonal antibody (mAb).1 Ideally, this chemical linker is stable to systemic metabolism, but cleavable within target cells such that it releases the cytotoxic agent selectively within the target cells, ie, after lysosomal degradation.2 The mAb also plays a part, selectively targeting specific surface antigens that are present on tumour cells. Ideally, the selected antigen target is only expressed by the diseased cells and shows limited expression in healthy cells.1
ADCs represent a fast-growing segment of the pharmaceutical market”
ADCs represent a fast-growing segment of the pharmaceutical market because of their potential to treat oncology indications with high unmet patient need. However, early attempts to advance ADCs through the clinic and onto the market were fraught with challenges due to their complex makeup and cytotoxic effects. Over the years, many advancements have been made across ADC discovery, design, development, manufacturing and clinical trial design, leading to improved clinical outcomes. New clinical successes and an influx of recent approvals have led to increased interest in the industry.3 Today, pharmaceutical companies small and large continue to advance ADC technology with the help of partnerships and collaborations to bring ADCs to the clinic and market. A list of currently approved ADCs is presented in Table 1.
Early challenges gave way to improved ADC designs
Initially, researchers focused solely on the antibody and protein targets without meaningfully considering the ADC as a whole. Consequently, first‑generation ADCs encountered several significant issues, including poor systemic linker stability, short circulating resident times, low tumour internalisation, low loading capacity, off-target toxicity, drug resistance and immunogenicity.3 More recently, ADC programmes have focused on more specific mAbs with better connectivity to the linker and better linkers, together with an optimal selection of potency and payload of the cytotoxic drug.5
Modifying those sites on the mAb where the linker is attached to the antibody, is an important research goal. Historically, most ADCs had variable numbers of linkers attached to each antibody, ie, heterogeneous linkages. It was challenging to optimise the drug-to-antibody ratio (DAR) for each ADC and to ensure homogeneity in ADC manufacturing batches. Manufacturability was also a key concern, as scale up and batch-to-batch consistency can vary for these complex molecules. Achieving high enough titres of antibody expression is always a goal and maintaining high titres as manufacturing is scaled from pre-clinical to clinical to commercial impacts the cost of goods of the product. In contrast, creating homogenous ADCs where each antibody is attached to the same number of linker groups, improves specificity. One way to do this is to use site-specific conjugation to direct the attachment of the linker to a specific location on the antibody. Catalent developed SMARTag® site-specific bioconjugation technology to generate homogenous linkages.6 A string of six amino acids are encoded into the antibody, which is recognised by a specific enzyme that converts a cysteine residue within that sequence into a formylglycine residue. The formylglycine contains an aldehyde functional group that serves as a handle for site‑specific conjugation. The result is the formation of a highly stable C-C bond connecting the antibody to the linker group.5 TRPH-222 is a CD22-directed ADC developed using SMARTag® technology and currently being advanced through Phase I clinical trials by Triphase Accelerator Corporation for patients with relapsed/refractory non-Hodgkin’s lymphoma (NCT03682796). In Triphase’s announcement7 (of their interim Phase I results), they disclosed that they have been able to dose patients up to 10 milligrams per kilogram, which is a significantly higher dose than is typically observed for ADCs in its class, which carry a maytansine payload attached through a non-cleavable linker.
Diverse strategies for engineering of ADC designs
There is ongoing debate about drug payload and toxicity. Currently, the most common payload to antibody stoichiometry is four drug molecules per ADC. The efficacy can be enhanced by having higher antibody loading of a less toxic payload, which can translate into improved ADC tolerability. For example, Trodelvy® carries eight molecules of the moderately potent topoisomerase inhibitor payload, SN-38, per antibody. Similarly, Enhertu® carries eight molecules of the related topoisomerase inhibitor payload, exatecan.
Antibody to payload stoichiometry is not the only area of innovation for ADCs. The adoption of new payloads is also being credited for recent promising clinical results. New payload classes include the topoisomerase inhibitors mentioned above (also utilised by Trodelvy®) and toll-like receptor (TLR) agonists. While it remains to be seen whether pyrrolobenzodiazepines (PBDs) will become a favoured payload, another DNA alkylating payload, indolinobenzodiazepine dimer (IGN), has also been investigated in some ADCs.8,9 Scientists are getting a better handle on which type of payload is effective against each specific type of cancer. For instance, there is a rise in solid tumour applications with ADCs owing to the emergence of use of a variety of different payloads that might be more effective against solid compared to hematopoietic tumours.
Antibody to payload stoichiometry is not the only area of innovation for ADCs”
In addition to achieving the optimal drug-to-antibody ratio (DAR) for each ADC and choosing the right payload, the location of the payload on the antibody is important for efficacy, stability and other pharmacokinetic and pharmacodynamic considerations. The importance of payload location and the need to optimise DAR in order to widen the therapeutic window also provide reasons to leverage site-specific bioconjugation technologies when developing ADCs.
The payload-linker combination is also important. Trodelvy® has a novel labile carbonate linker that can be hydrolysed in acidic or basic environments. The linker also contains hydrophilic PEG (propylene glycol) units, which boost aqueous solubility, and a lysine molecule that prevents the ADC from aggregation, which can prompt increased immunogenicity. Other linkers contain hydrophilic glucuronates, which can be cleaved by β-glucuronidase enzymes in the lysosomes, ie, targeted lysosomal degradation.5 Conjugate stability – keeping the payload on the antibody – is an important consideration, because if the linker is unstable in the bloodstream, there is a risk that the cytotoxic payload is released prematurely, before it reaches its target, potentially causing local or even systemic toxicity. TRPH-222 utilises unique linker chemistry with the goal of improving clinical and patient outcomes. This linker, used in the clinical-stage anti-CD22 ADC, has shown the ability to resist efflux out of the tumour cell, which provides the potential to overcome drug resistance.6,10
In some cases, a cytotoxic drug released into a target cell can also diffuse into neighbouring cells and tissues, the so-called ‘bystander’ effect.11 This effect can be beneficial; for example, in cases where tumours heterogeneously express the target antigen, the payload can be delivered to antigen-negative tumour cells adjacent to antigen-positive tumour cells. However, healthy tissues in proximity to targeted tumour cells can also be damaged as a result of local toxin diffusion away from the tumour. In contrast, there is a better kill-rate of cancer cells with more toxic drugs, although the side effect profile of the drug may be less beneficial. Higher potency compounds such as PBDs have been investigated for their potential as ADC payloads after promising preclinical results. However, some PBD-containing ADCs have failed in late-stage, pivotal trials, including Seagen’s vadastuximab talirine® and AbbVie’s rovalpituzumab tesirine®. The withdrawal of these drugs in late-stage trials has likely led to decreased interest in PBDs as a payload, although there are some companies that are currently still progressing PBD-based ADCs through clinical trials. Prominent among these are ADC Therapeutics, which has filed a Biologics License Application (BLA) for approval of their CD19-targeted drug, loncastuximab tesirine®, for the treatment of relapsed/refractory diffuse large B-cell lymphoma.12 If approved, it would represent the first ADC bearing this highly potent class of payloads.
Key analytical challenges
Analytical testing of ADCs is critical to development and manufacturing, as with any drug. But testing of ADCs can present additional challenges given the complexity of the molecules. For ADCs made using heterogeneous conjugation approaches, determining the location and number of conjugated payloads can be challenging when trying to characterise the molecule. As another example, the testing of free drug toxin levels in an ADC preparation is non-trivial. Such assays are commonly performed during release and stability to ensure that free drug toxin remains at safe levels. However, polymeric labware that is typically considered acceptable for the storage and handling of biologics can leach compounds that have hydrophobicity and UV absorption profiles that are similar to those of the free drug. Therefore, for typical drug substance and drug product storage, it is important to ensure that extractables and leachables will not interfere with free drug analysis.
Clinical trial design considerations
Improved knowledge of clinical study design is another area that is likely to result in further near-term approvals. Examples include how tumour antigen expression levels are assessed and used to monitor for early efficacy signals among the various tumour histologies in ‘basket trials’ to identify indications that are particularly responsive to ADC treatment. Clinical study design is especially important given that all but two ADCs approved to date have received one or more accelerated approval or review designations. The potential for ADCs to demonstrate substantial improvement over available therapies or to address unmet needs make them prime candidates for Fast Track Designation, Breakthrough Therapy, Priority Review or other accelerated pathways to approval. Improved clinical study design, as well as ability to efficiently scale manufacturing for clinical supply, are key to help ADC programmes meet the expedited timelines typically observed in programmes that receive these designations.
Recent successes and future outlook of ADCs
ADCs are becoming ever more useful tools in oncology and haematology. There are over 100 ADCs being currently evaluated in clinical trials.2,13 Notably, five ADCs have been approved in the past two years (Table 1) and the increased interest in the ADC market can be measured in part by the increased number of new ADCs entering the clinic and feeding the early-stage ‘funnel’. While the early-stage pipeline is heavily driven by small and virtual biotechs, mid- to large biopharma have taken note of these recent successes in the clinic. Gilead announced their agreement to acquire Immunomedics in September 2020,14 five months after Immunomedic’s ADC Trodelvy® was approved for metastatic triple-negative breast cancer.15 Merck was also very active in the ADC space the past year, entering into a licensing dealwith Seattle Genetics for a metastatic breast cancer ADC in September,16 one month after the approval of Blenrep®,17 which was developed and commercialised by GlaxoSmithKline using Seagen’s proprietary technology. Two months after the licensing deal with Seagen, Merck agreed to acquire VelosBio,18 whose lead candidate is a clinical-stage ADC for the treatment of patients with haematologic malignancies and solid tumours.
In addition to their potential as a monotherapy, ADCs are showing promising clinical results as complimentary combination agents, for example with checkpoint inhibitors. Studies are finding that the ADC creates a more favourable tumour microenvironment, within which the checkpoint inhibitor can be more effective. There is increased interest and growth in the use of combination treatments in this space; a Phase III trial is underway investigating enfortumab vedotin-ejfv (Padcev®) in combination with pembrolizumab (Opdivo®) to treat locally advanced or metastatic urothelial cancer (NCT04223856).
While the majority of ADC clinical trials are targeted toward oncology indications, ADCs will also likely expand out beyond cancer indications into other areas, such as autoimmune disorders. Belantamab mafodotin (Blenrep®) and Brentuximab vedotin (Adcetris®), which target antigens expressed on immune cell populations, are currently in clinical trials for several non-cancer indications. Other ADC candidates are in clinical trials for rheumatoid arthritis and infectious diseases, among other indications. The expansion beyond oncology indications might open the door to using non‑cytotoxic payloads, including other therapeutic small molecules, proteins, nucleic acids and other molecule types. All told, the future looks bright for this therapeutic approach to address oncology indications and beyond.
About the authors
Stacey Treichler joined Catalent in 2017 and is currently the Group Product Manager – Biologics, supporting the drug substance, drug product, analytical and antibody-drug conjugate businesses. Prior to Catalent, Stacey spent five years as Product Manager and Senior Product Manager in the drug substance, medical device and molecular diagnostics industries, supporting and launching multiple products for bioprocessing, organ transplant and microbial testing. Stacey received a bachelor’s degree in Biology from Ursinus College and a PhD in Molecular Biology from Rutgers University.
Registered Trademarks
- Enhertu is a registered trademark of Daiichi Sankyo Company, Limited.
- Kadcyla and Polivy are registered trademarks of Genentech USA, Inc.
- Trodelvy is a registered trademark of Immunomedics, Inc.
- Besponsa and Mylotarg are trademarks of Pfizer, Inc.
- Adcetris is a registered trademark of Seagen, Inc.
- Catalent and SMARTag are registered trademarks of Catalent Pharma Solutions, Inc.
- Blenrep is a registered trademark of Glaxo Group Limited.
- Padcev is a registered trademark jointly owned by Agensys, Inc. and Seagen, Inc.
- Opdivo is a registered trademark of Bristol-Myers Squibb Company.
References
- Walko CM, West HJ. Antibody Drug Conjugates for Cancer Treatment. JAMA Oncol. 2019; 5(11):1648. doi:10.1001/jamaoncol.2019.3552.
- Chau CH, Steeg PS, Figg WD. Antibody–drug conjugates for cancer. The Lancet Therapeutics, 2019; 394(10200): P793-P804.
- Nejadmoghaddam MR, Minai-Tehrani A, Ghahremanzadeh R, Mahmoudi M, et al. Antibody-Drug Conjugates: Possibilities and Challenges. Avicenna J Med Biotechnol. 2019; 11(1): 3-23.
- Development status based on AdisInsight (http://adisinsight.springer.com), accessed on March 14, 2021
- Halford B. A new generation of antibody-drug conjugates for cancer patients. C&EN. 12 Apr 2020; 98 (114). https://cen.acs.org/biological-chemistry/cancer/new-generation-antibody-drug-conjugates/98/i14. Accessed on 27 December 2020.
- Drake PM, Albers AE, Baker J, et al. Aldehyde Tag Coupled with HIPS Chemistry Enables the Production of ADCs Conjugated Site-Specifically to Different Antibody Regions with Distinct in Vivo Efficacy and PK Outcomes. Bioconjugate Chem, 2014; 25:7:1331-1341.
- Triphase Accelerator Announces Positive Interim Results of Phase 1 Trial of TRPH-222 in Patients with Relapsed/Refractory Non-Hodgkin Lymphoma. Oral Presentation to the American Society of Hematology (ASH) 2020 Annual Meeting, 07 Dec 2020. Accessed on 16 March 2021.
- Cavallo J. SABCS 2019: [Fam-] Trastuzumab Deruxtecan in Patients with Pretreated HER2-Positive Metastatic Breast Cancer. The ASCO Post. 2019. https://www.ascopost.com/news/december-2019/t-dxd-in-patients-with-pretreated-her2-positive-metastatic-breast-cancer/. Accessed on 16 March 2021.
- Gingrich J. How the Next Generation Antibody Drug Conjugates Expand Beyond Cytotoxic Payloads for Cancer Therapy, j. Antibidy Drug conjugates. 2020. https://www.adcreview.com/articles/how-the-next-generation-antibody-drug-conjugates-expands-beyond-cytotoxic-payloads-for-cancer-therapy/ Accessed on 16 March 2021. .
- Drake PM, Carlson A, McFarland JM, et al. CAT-02-106, a Site-Specifically Conjugated Anti-CD22 Antibody Bearing an MDR1-Resistant Maytansine Payload Yields Excellent Efficacy and Safety in Preclinical Models. Molecular Cancer Therapeutics. 2018; 17: 161–168.
- Staudacher AH, Brown MP. Antibody drug conjugates and bystander killing: is antigen-dependent internalisation required? Brit J Cancer, 2017; 117: 1736-1742.
- ADC Therapeutics Announces FDA Accepts Biologics License Application and Grants Priority Review for Loncastuximab Tesirine for Treatment of Relapsed or Refractory Diffuse Large B-cell Lymphoma. 20 November 2020. https://www.businesswire.com/news/home/20201120005201/en/ADC-Therapeutics-Announces-FDA-Accepts-Biologics-License-Application-and-Grants-Priority-Review-for-Loncastuximab-Tesirine-for-Treatment-of-Relapsed-or-Refractory-Diffuse-Large-B-cell-Lymphoma. Accessed on 16 March 2021.
- ADC Review. Resources for Clinicians. https://www.adcreview.com/resources-for-for-clinicians/. Accessed on 16 N=March 2021.
- Gilead Sciences to Acquire Immunomedics. 13 September 2020. https://www.gilead.com/news-and-press/press-room/press-releases/2020/9/gilead-sciences-to-acquire-immunomedics. Accessed on 16 March 2021.
- FDA approves GSK’s BLENREP (belantamab mafodotin-blmf) for the treatment of patients with relapsed or refractory multiple myeloma. 06 August 2020. https://www.gsk.com/en-gb/media/press-releases/fda-approves-gsk-s-blenrep-belantamab-mafodotin-blmf-for-the-treatment-of-patients-with-relapsed-or-refractory-multiple-myeloma/. Accessed on 16 March 2021.
- Seattle Genetics and Merck announce two strategic collaborations in oncology. 14 September 2020. https://www.merck.com/news/seattle-genetics-and-merck-announce-two-strategic-oncology-collaborations/. Accessed on 16 March 2020.
- FDA Approves New Therapy for Triple Negative Breast Cancer That Has Spread, Not Responded to Other Treatments. 22 April 2020. https://www.fda.gov/news-events/press-announcements/fda-approves-new-therapy-triple-negative-breast-cancer-has-spread-not-responded-other-treatments. Accessed on 16 March 2021.
- Merck to acquire VelosBio, 05 November 2020. https://www.merck.com/news/merck-to-acquire-velosbio/. Assessed on 16 March 2020.
Issue
Related topics
Antibodies, Biologics, Biopharmaceuticals, Drug Development, Drug Safety, Drug Targets, Research & Development (R&D), Therapeutics
Related organisations
AbbVie, Bristol-Myers Squibb (BMS), Catalent, Daiichi Sankyo, Genentech Inc, Glaxo Group Limited, Immunomedics Inc., Pfizer Inc., Seagen
Related drugs
Adcetris (brentuximab vedotin), Besponsa, Blenrep (belantamab mafodotin-blmf), Enhertu (trastuzumab deruxtecan), Mylotarg, Opdivo (nivolumab), PADCEV, Polivy (polatuzumab vedotin), trastuzumab emtansine (Kadcyla), Trodelvy (sacituzumab govitecan-hziy)