What is label-free screening and why use it in drug discovery?
Posted: 18 December 2012 |
In the journey of a molecule from its origins in a compound library to candidate drug status, a large variety of profiling must occur to define activity, selectivity, potency, adverse effects, pharmacology and in vivo efficacy. Advances in biophysical methods that can analyse drug interactions with a molecular target, a whole cell, or even ex vivo tissue have enabled many of these studies to be carried out without the need for reporter-based or ‘labelled’ assays. Label-free screening in high-throughput mode can be used as a pathway independent screening tool with whole cells, or in low-throughput mode with individual receptors to define interaction kinetics and thermodynamics. We highlight advances in optical and impedance-based biosensors, and examine their utility and suitability for various stages of the drug discovery process.
In the early to mid 20th century, drug discovery was a far more productive industry, and more drugs were launched per Pharma employee than today. The regulatory pathway that pre-empted the launch of a new drug was concise and easy to understand, and applications were dealt with expeditiously with a fraction of the supporting data required today. The process of discovery was also very different; it was driven largely by individuals in small teams who were prepared for serendipity, or by individuals with a very clear, defined hypothesis who drove rational drug design. Screening technologies could be summed up on one or two pages of a review; a dozen or so primary assays, some basic biochemistry to define ligand mode of action, perhaps some live cell work and a proof of concept demonstration in vivo…
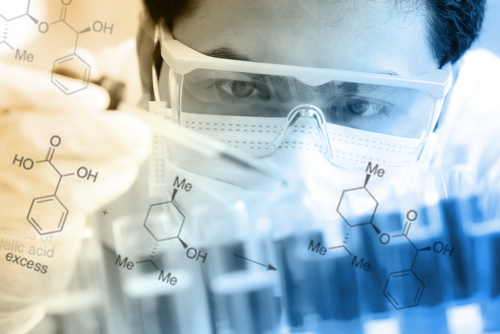
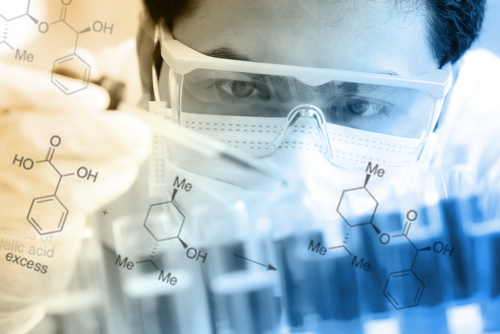
In the journey of a molecule from its origins in a compound library to candidate drug status, a large variety of profiling must occur to define activity, selectivity, potency, adverse effects, pharmacology and in vivo efficacy. Advances in biophysical methods that can analyse drug interactions with a molecular target, a whole cell, or even ex vivo tissue have enabled many of these studies to be carried out without the need for reporter-based or ‘labelled’ assays. Label-free screening in high-throughput mode can be used as a pathway independent screening tool with whole cells, or in low-throughput mode with individual receptors to define interaction kinetics and thermodynamics. We highlight advances in optical and impedance-based biosensors, and examine their utility and suitability for various stages of the drug discovery process.
In the early to mid 20th century, drug discovery was a far more productive industry, and more drugs were launched per Pharma employee than today. The regulatory pathway that pre-empted the launch of a new drug was concise and easy to understand, and applications were dealt with expeditiously with a fraction of the supporting data required today. The process of discovery was also very different; it was driven largely by individuals in small teams who were prepared for serendipity, or by individuals with a very clear, defined hypothesis who drove rational drug design. Screening technologies could be summed up on one or two pages of a review; a dozen or so primary assays, some basic biochemistry to define ligand mode of action, perhaps some live cell work and a proof of concept demonstration in vivo.
A paradigm shift then occurred in the 1980s. The pharmaceutical industry became more profitable and globalised. Innovation was distributed from small, concen – trated ‘centres of excellence’ into discipline based silos: target validation, screening, medicinal chemistry, toxicology, pharmacology and clinical studies. In extreme cases, many of these silos were not aware of the ultimate fate of the molecules that passed from one centre of expertise to another. The surging profit margins in Pharma also attracted different people with new ideas on how to make drug discovery more efficient. Management consultants looked at the slow, sometimes stochastic path from idea to molecule to drug, and derived numerical algorithms to improve productivity to deliver a faster drug pipeline. Many of these could be summarised as ‘make more molecules and screen more molecules against more targets to increase the chance of finding a drug’. In turn, this drove the development of combinatorial chemistry and high-throughput screening. Both approaches immediately limited the scope of discovery. Combinatorial chemistry needed to be high yielding, efficient and was only compatible with a limited sub-set of the gamut of rich solutionphase, technically challenging chemistry available. This directly led to a reduction in the median molecular diversity within compound libraries. Drug screening became focused on a limited sub-set of assays that exploited fluorescent or luminescence to report the action of a ligand. Cost, efficiency, and assay robustness metrics such as the Z-factor, became the driving criteria behind assay selection. In many cases, the relevance of the assay to the tissue or cell-state found in active disease was lost.
Drug action and response
During this time, a small sub-set of researchers and instrument developers were still interested in the maxim that for a drug to work, it first must bind to its receptor. Binding based assays were based primarily on radioactive pull-down formats with solubilised receptors, which required considerable care and preparation to achieve1. The advent of the SPR-based biosensors to quantify protein-protein inter – actions raised awareness of techniques that could report binding without radio- or fluorescent-labels2,3. This allowed us to study the real-time interaction between immobilised receptors and analytes, without labelling of the analyte; leading to the term ‘label-free’. Although originally designed as a method of determining affinities, advances in microfluidic delivery systems allowed kinetics (on and off rates of binding) to be measured, subsequently leading to new insight into compound action and ultimately new optimisation strategies. Some optical and acoustic based biosensor systems are able to make measurements directly in crude matrices such as growth media and serum. This has extended the range of applications of labelfree from characterisation of primary hits, through to pre-clinical profiling of lead compounds. Information on compound specificity, kinetics and affinity between a drugreceptor interaction can be inferred from binding rates and binding levels. As Paul Ehrlich rightfully stated, “Corpora non agunt nisi fixate”: a drug will not work unless it is bound (Paul Ehrlich; 1854-1915). This statement has guided many drug discovery programs to date, but more consideration is now being given to how and what biochemical and physiological mechanisms are involved in producing the biological response.
For example, it is entirely feasible that structurally similar molecules have similar binding affinities, however differ by the rate at which they dissociate from the receptor and therefore exhibit very different therapeutic effects in vivo. The orientation, the ability to induce a conformational change, reversibility, stimulation or blocking effects, are all factors that contribute to the effectiveness of a drug. Slight changes in the chemical structure of a compound can switch its mode of action from a stimulatory compound (agonist) to an inhibit – ory compound (antagonist). It has been proposed that drugs behave in ways other than the classical view of rapid equilibrium binding of drug to targets4. The usefulness of a drug and its therapeutic index can be accurately defined by optimal binding modes. Label-free techniques can aid in the optimisation of such mechanisms and in turn further our understanding of drugs with respect to predictive pharmacodynamics, binding5, off target effects / toxicity6 and a plethora of other properties that lead to the identification of superior drug properties in one compound over another.
From the 1980s, when the first commercial label-free biosensors were developed, their value in research and development across multiple disciples from primary research to translational research has been demonstrated. The throughput capacity of these instruments has improved immensely from the days of manual, laborious low-throughput assays. With the help of advanced liquid handling, it is now easy to run a large number of samples with relatively small sample volumes in an auto – mated manner, even in the case of classical techniques such as isothermal titration calorimetry (ITC)8. Advances in instrument components and better data analysis has led to high-throughput non-fluorescent (white light) high-content cell-based assays9,10. Mass spectrometry is now firmly established in drug discovery and compound profiling11. Nuclear magnetic resonance (NMR) methods have enabled everyday applicability in drug discovery for compound and drug fragment screening against soluble targets12. Its use and value has been mostly appreciated in the structural biology field, but the implications of NMR for structure aided drug design to improve hit validation and optimisation are large. In alliance with the need for high-throughput screening, fragment based screening approaches using NMR have become popular, where important information can be gained from key interactions of small fragments to the active site of the receptor of interest13.
We now have a better insight into the value of drug binding kinetics and target residence time (the percentage of time for which the target binding site is occupied by drug). Copeland et al.7 have noted that in lab-based, equilibrium binding experiments, the receptor is exposed to an invariant concen – tration of ligand, which is normally in excess compared to the molar amount of molecular receptor. Here the drug-target residence time is related to the equilibrium dissociation constant. However, in a dynamic in vivo situation, the concentration of ligand varies with time accord – ing to dosing regime, uptake, bioavailability, reservoir deposition, metabolism, excretion and many other factors. With such variability in drug concentration, the molecular action of a molecule can be better described not by the in vitro affinity for the target, but rather by the association rate constant or on-rate (kon) and, more importantly the dissociation rate constant, or off-rate (koff) of the receptor–ligand complex, which is often stated in terms of the half-life as a direct measure of drug residence time. Copeland et al.7 hence propose that in vitro measurement of the dissociative half-life, which may be accurately measured by label-free techniques such as SPR (surface plasmon resonance), may be a crucial metric of compound optimisation, and could be a key indicator of in vivo duration of efficacy.
Pathway-independent screening with label-free using whole cells
The development of label-free technologies that allowed for the detection of cellular events in whole cells was a welcome introduction in compound screening. These technologies have exploited cell impedance and resonant waveguide grating to allow for the detection of cell signalling events, morpho – logical changes and proliferation, amongst other novel applications.
The immediate impact for these tech – nologies has been seen in GPCR screening, reflected by the large number of publications in this area. GPCRs have been and continue to be a ‘hot target’ for the development of thera – peutics14. However, traditional validation and identification of hits encompassed the use of transfected cell lines, which were not really an ideal reflection of disease relevant cell types. The insensitivity of label-based assays was a key driver for the use of transfected cell lines overexpressing the receptor of interest. Due to costs and other limitations, compounds were screened in a label-based selective assay, usually the cheapest, with the highest throughput. This selection criterion already biased the hits identified, as contradictory to previous beliefs, not all compounds trigger a full cell signalling response in the cell15. Some compounds are biased to one signalling pathway over another and these hits could prove to be valuable leads in their own right, by reducing potential nonspecific signalling events while maintaining their full therapeutic potential. The label-free technologies were thus welcome, particularly in this area of science, as they provided a superior sensitive platform for screening compounds in native cells, without the use of labels, where entire cell responses could be captured in an unbiased manner. Since their release, comparative studies between native and transfected cell lines have highlighted the flaws in the traditional screening paradigms, further supporting the notion that label-free technologies provide more biologically relevant data16.
The development from 96 to 384 to 1536 well formats has ensured the continual use of these technologies in screening. More effort is now being made into understanding the extra data these platforms provide with respect to what signalling events are detected, or what other toxicity information can be gained per assay. The label-free technologies meeting in Amsterdam in November 2012 covered the latest and emerging developments in label-free detection systems, their underlying technology principles and end-user case studies that reveal the power and limitations of label-free in all areas of drug discovery17. The potential of label-free approaches to complement or even displace other technologies has never been higher.
References
- Bylund, D. B., and M. L. Toews. 1993. Radioligand binding methods: practical guide and tips. Am J Physiol 265:421-429
- Homola, J., S. S. Yee, and G. Gauglitz. 1999. Surface plasmon resonance sensors: review. Sensor Actuat BChem 54:3-15
- Cooper, M. A. 2002. Optical biosensors in drug discovery. Nat Rev Drug Discov 1:515-528
- Swinney, D. C. 2004. Biochemical mechanisms of drug action: what does it take for success? Nat Rev Drug Discov 3:801-808
- Myszka, D. G. 2004. Analysis of small-molecule interactions using Biacore S51 technology. Anal Biochem 329:316-323
- Hu, Q., D. D. Zhang, L. Wang, H. Lou, and D. Ren. 2012. Eriodictyol-7-O-glucoside, a novel Nrf2 activator, confers protection against cisplatin-induced toxicity. Food Chem Toxicol 50:1927-1932
- Copeland, R. A., D. L. Pompliano, and T. D. Meek. 2006. Drug-target residence time and its implications for lead optimization. Nat Rev Drug Discov 5:730-739
- Peters, W. B., V. Frasca, and R. K. Brown. 2009. Recent developments in isothermal titration calorimetry label free screening. Comb Chem High Throughput Screen 12:772-790
- Paveley, R. A., N. R. Mansour, I. Hallyburton, L. S. Bleicher, A. E. Benn, I. Mikic, A. Guidi, I. H. Gilbert, A. L. Hopkins, and Q. D. Bickle. 2012. Whole organism high-content screening by label-free, image-based Bayesian classification for parasitic diseases. PLoS Negl Trop Dis 6:e1762
- Kleinhans, R., M. Brischwein, P. Wang, B. Becker, F. Demmel, T. Schwarzenberger, M. Zottmann, P. Wolf, A. Niendorf, and B. Wolf. 2012. Sensor-based cell and tissue screening for personalized cancer chemotherapy. Med Biol Eng Comput 50:117-126
- Feng, W. Y. 2004. Mass spectrometry in drug discovery: a current review. Curr Drug Discov Technol 1:295-312
- Saio, T., K. Ogura, K. Shimizu, M. Yokochi, T. R. Burke, Jr., and F. Inagaki. 2011. An NMR strategy for fragment-based ligand screening utilizing a paramagnetic lanthanide probe. J Biomol NMR 51:395-408
- Pellecchia, M., I. Bertini, D. Cowburn, C. Dalvit, E. Giralt, W. Jahnke, T. L. James, S. W. Homans, H. Kessler, C. Luchinat, B. Meyer, H. Oschkinat, J. Peng, H. Schwalbe, and G. Siegal. 2008. Perspectives on NMR in drug discovery: a technique comes of age. Nat Rev Drug Discov 7:738-745
- Mason, J. S., A. Bortolato, M. Congreve, and F. H. Marshall. 2012. New insights from structural biology into the druggability of G protein-coupled receptors. Trends Pharmacol Sci 33:249-260
- Scholten, D. J., M. Canals, M. Wijtmans, S. de Munnik, P. Nguyen, D. Verzijl, I. J. de Esch, H. F. Vischer, M. J. Smit, and R. Leurs. 2012. Pharmacological characterization of a small-molecule agonist for the chemokine receptor CXCR3. Br J Pharmacol 166:898-911
- Halai, R., D. E. Croker, J. Y. Suen, D. P. Fairlie, and M. A. Cooper. 2012. A Comparative Study of Impedance versus Optical Label-Free Systems Relative to Labelled Assays in a Predominantly Gi Coupled GPCR (C5aR) Signalling. Biosensors 2:273-290
- Halai, R., and M. A. Cooper. 2012. Using label-free screening technology to improve efficiency in drug discovery. Expert Opin Drug Discovery 7:123-131
About the authors
Professor Matthew Cooper completed his PhD in Australia in 1995 and then spent 13 years in the UK, first at the University of Cambridge, then nine years in start-ups and biotechnology companies. He returned to Australia in 2009 as a NHMRC Australia Fellow, at The Institute for Molecular Bioscience at The University of Queensland. He has consulted with Private Equity Investment, Pharmaceutical, Biotechnology and Diagnostics companies, was Managing Director of Cambridge Medical Innovations (part of Alere Inc.) and CSO of Akubio. He has published widely on the development and use of label-free technologies in drug discovery and has edited two books in this field: Label-Free Technologies for Drug Discovery (Wiley Blackwell, 2011) and Label-free Biosensors: Techniques and Applications (Cambridge University Press, 2009).
Dr. Reena Halai is a Research Officer at the Institute for Molecular Bioscience. She received her BSc degree in Biochemistry from Kings College London, during which she completed her placement year at GlaxoSmithKline in the Gene Expression and Protein Biochemistry Department. She then went onto work for the oncology department at UCB Pharmaceuticals before commencing her Ph.D. with Prof. D. Craik at the Institute for Molecular Bioscience. Reena has numerous papers in peer reviewed journals and is now part of the Cooper Group, where her current research interest is label-free technologies.