Implementing Near Infrared Spectroscopy as a PAT tool in the biopharmaceutical industries
Posted: 21 July 2007 | | No comments yet
Biopharmaceuticals are the fastest growing sector of the pharmaceutical industry[1]; with monoclonal’s being the key biopharmaceutical products representing a significant proportion of the current business focus. It is anticipated that this new generation of biopharmaceuticals will revolutionise clinical medicine over the next 5-10 years.
Biopharmaceuticals are the fastest growing sector of the pharmaceutical industry[1]; with monoclonal’s being the key biopharmaceutical products representing a significant proportion of the current business focus. It is anticipated that this new generation of biopharmaceuticals will revolutionise clinical medicine over the next 5-10 years.
Biopharmaceuticals are the fastest growing sector of the pharmaceutical industry[1]; with monoclonal’s being the key biopharmaceutical products representing a significant proportion of the current business focus. It is anticipated that this new generation of biopharmaceuticals will revolutionise clinical medicine over the next 5-10 years.
The Food and Drug Administration (FDA) announced their guidance document on Process Analytical Technology (PAT) in 2004[2]; this prompted many pharmaceutical companies to re-evaluate their future requirements for bioprocess analysis. The PAT initiative specifically encourages the development of improved process monitoring technology in the manufacturing environment. This has effectively led the investigation of a number of novel technologies in a biomanufacturing context, and much attention has been focused on near infrared (NIR) spectroscopy. Potentially, NIR offers the prospect of real-time control of the physiology of cultured cells in fermenters; leading to marked improvements in authenticity, purity and production efficiency.
For mammalian cell culture processes, in situ monitoring of the process variables/parameters is generally favoured above off-line analysis, as monitoring off-line would require a manual extraction step which is a potential threat to the process asepsis. Therefore, NIRS offers significant opportunities for analysis and control of process parameters, as measurements can be made in situ; offering the additional prospect of multiple vessels being monitored with the use of fibre optics. The ability to multiplex NIRS has created new possibilities for bioprocess monitoring.
Today’s competitive industrial environment requires manufacturers to continuously strive for improved product quality while reducing manufacturing costs; NIRS is particularly well suited to process analysis, helping to achieve these goals. One major advantage of NIRS is that it can supply multianalyte information at a rapid rate with minimal effort in sample preparation. Thus, it is readily accessible to a process operator (as opposed to a skilled analyst) and can be implemented on a routine basis. The improved quantity and quality of information can be used to optimise process control, leading to higher productivity, decreased batch to batch variability, and potentially improved product quality.
The purpose of this article is to highlight the reasons for the increasing acceptance of NIR as a PAT tool. The article will also focus on the applicability of NIR within the biopharmaceutical industry based on the author’s experience on multiplexing in situ NIRS to monitor Chinese Hamster Ovary (CHO) cell cultures.
NIRS Background
William Herschel discovered the NIR region in 1800, but the potential of NIRS was not realised until the work of Karl Norris in the 1960s. With advances in computing technology, software, optics etc; it is widely used today for the determination of key components from such diverse fields as agriculture and petrochemical, as well as having recently been applied to the bedside monitoring of the new born brain3.
NIR encompasses the narrow spectral region of the electro-magnetic spectrum between 700-2500 nm. At the molecular level, most NIR spectral information originates from vibrations involving hydrogen bonds such as C-H, O-H, N-H, S-H, etc, which are commonly encountered in bio-molecules. Therefore, almost all major chemical constituents are measurable by NIRS.
In seeking to apply NIR as a PAT tool, vigilant consideration must be given to what is to be monitored, and where that monitoring can be effectively completed to provide information that can be acted upon. Generally, a guiding principle to bioprocess monitoring might be “to disturb the sample collected as insignificantly as possible”. The more the sample is disturbed by the measuring system, the more foreign objects are introduced into the measurement. These objects must then be compensated for in order to correlate well with the spectral information. Therefore, there is a pressing need for a feasible on-line monitoring tool.
Advances in NIR probes and analyser/software technology have made many measurements possible that would have previously been impractical; the technology has now matured to the point where it is routinely used in laboratory environments by non-spectroscopists. Among the available on-line techniques, NIR offers a number of attractive features that are suitable for the purpose including: simplicity and rapidity of measurement, flexibility in application and inherent potential to include process variations through chemometrics.
As mentioned previously, FDA led PAT is optimistic that it can encourage pharmaceutical industries to improve the state of their manufacturing technology by reducing the regulatory burden that currently hinders continuous process development. NIR appears to be one of the technologies that shows promise for improving manufacturing in the biotechnology industry. NIR is now being rapidly used in the herbicide, fungicide and the pharmaceutical industries for moisture measurement, raw material identification, quantification of the polymorphic content of a pharmaceutical compound, testing of tablets for content uniformity and concentration etc; These processes have been difficult to monitor on-line in the past.
The measurement of solid samples and highly scattering slurries is generally made through a reflectance probe interfaced to the process or to the sampling window. For liquid systems, both transmission and transflection measurements are applicable. However, the optimal pathlength which provides relevant information and is best suited to the process must be determined.
Instrumentation: fibre optic NIRS
In comparison to mid-IR, NIR instruments are based on simpler optics and are much cheaper to construct. A typical conventional NIR spectrometer includes a tungsten- halogen filament lamp that provides the radiation source, a monochromator for selecting wavelengths, a sample cell and a detector to detect the signal that is received after interaction with the sample.
The NIR absorbances are typically 100-1000 times weaker than mid-IR absorbances, therefore, NIR radiation must traverse through 100-1000 times more material to attain useful spectrum. Thus, a wider pathlength of typically 0.2-5 cm could be utilised which in turn increases sensitivity. In addition, longer optical fibre cables are feasible and durable window/lens materials are available, making NIR more practical and appealing from the aspect of real-time monitoring. Bright sources and low noise detectors are also available in the NIR region. Therefore, a large signal to noise (S/N) ratio can be achieved and greater S/N ratio translates into better concentration sensitivity for measurement.
Most biotechnological processes have strict requirements to maintain the system sterility (monosepsis). Any contact between the reactors’ content and the outside environment represents a grave risk to the sterility of the process; the NIR probe must therefore be steam sterilisable, as a result, only physically robust sensors can be used in the reactor vessels. Today, the NIR probes manufactured by probe suppliers such as Precision Sensing, UK, provide 12 mm and 25 mm stainless steel probes as depicted in Figure 1; custom designed for application in autoclavable/steam in place (SIP) bioreactors respectively, with sapphire window material, detachable protective caps and detachable mirrors (Figure 1C); gold plated to resist the high temperature and pressure of the reactor vessel.
NIR systems manufacturer, Bruker Optics, provides an internal multiplexer for up to six fibre optics channels enabling the use of both conventional, and specially designed probes. Implementation of the fibre-optic technology allows the flexibility to monitor a number of reactor vessels simultaneously or from different regions in the same reactor vessel to a common analyser, resulting in both efficient and enhanced process monitoring.
Real time process monitoring is currently being achieved by three main types of fibre optic NIR probes: transmittance, reflectance and transflectance. The probes are usually constructed of high-grade stainless steel, titanium, or even tantalum, to provide enough physical and chemical robustness to withstand the process stream.
The seal between the probe window and the probe body is crucial and is generally made up of a form of fluroploymer “O” ringed compression fitting or a mixed-metal brazed joint. The window material used in the probes generally depends on the technique being used. For NIR, visible, Raman, and other visible laser spectroscopies, sapphire is the mostly commonly used material. The other commonly used material is quartz (NIR/visible/UV) and silica glass (Raman). The optical fibres connecting the probes to the analyser are generally made up of silica or quartz. The optical fibres generally have a core diameter of 50-100µm to minimise their interaction with the radiation they transmit. However, the fibres should be handled carefully to ensure that they are not bent as micro-fractures start generating through the fibre matrix over time, and this may lead to a complete loss of transmission. The fibres are therefore protected with a coating, for example; PVC, polyamide, direct metal, external stainless braid etc.
Having discussed the instrumentation of fibre optic NIRS, the next topic for consideration would be the mathematical modelling involved in correlating the spectral information with reference assay. For complex matrices as those observed in fermentation fluids, Partial Least Square (PLS) and Principal Component Analysis (PCA) are the most commonly used techniques, mostly because NIR has characteristically broad absorption bands, and peaks from the analyte of interest can be concealed easily within much broader peaks resulting from other analytes.
There are a number of commercially available software packages which can be used to correlate the spectral information with the reference data such as: Vision, SIMCA, OPUS, Unscrambler and Matlab. Multivariate data analysis of NIR and process data demonstrates a great deal of potential in bioprocess understanding and optimisation.
Bioprocess monitoring
The application of NIRS in bioprocess monitoring is more challenging than in other areas of science as the process environment is constantly changing; it involves living entities and the nature of the fermentation itself can often be viscous and heterogeneous. Historically, conventional NIR (single probe) calibration techniques have been limited in their approach with modelling based on data collected from a single reactor failing to incorporate the reactor variability, an essential parameter when developing a robust model. With multiplex technology, the user can incorporate the reactor variability in the models, as multiple reactors can be monitored simultaneously using a single analyser reducing the time generally required, when using the conventional calibration technique.
Arnold et al.4 reported the in situ monitoring of animal cell culture processes using the conventional single probe model and developed successful models for the key analytes of interest. Therefore, implementing multiplexing fibre optic NIR spectroscopy to monitor such a bioprocess should be relatively straightforward as mammalian cells tend to be simpler processes with low biomass, operated without vigorous mixing or sparging and small but insignificant rheological changes. The growth media formulations are homogenous and do not contain any significant solids or lipid components; there has been no evidence of probe fouling in mammalian cell culture vessels. Furthermore, most developmental processes are generic in nature, this provides the additional prospect of investigating the generic predictive ability of the models i.e. models based on a particular cell line can be used to monitor a different cell line.
However, with the multiplex technology a number of probes, mirrors and channels are implemented to monitor the bioprocess and this in itself may have an effect on the quality of spectra collected owing to the optical variability of the different components (probes, mirrors, channels) of the NIR system. This issue has been addressed in a previous finding by the authors own group5, as well as the ranking of optical variability of the NIR components via a systematic characterisation of the 3 key factors, namely: probes, mirrors and channels; Inferring that probe optical differences contribute most variance to signal. Nonetheless, they could build successful models using the multiplex systems which were comparable to the conventional single probe models giving high correlation coefficient values and lower errors of prediction.
An additional advantage of multiplexing includes model transferability as depicted in Figure 2: models which are based on small scale reactors could be exercised to monitor large scale reactors. Broadly, fermentation facilities are not designed according to scale up criteria and the process conditions in the culture vessels may differ significantly, therefore, successful scale up in most cases is not the result of a conclusive and straight lined experimental strategy, but rather an outcome of a separate process development and optimisation on each scale. This necessitates the need to conduct screening and optimisation studies at a small scale to identify the key variables which affect process performance, quality and productivity, as well as implementing the outcome of optimisation studies on to the large scale vessels.
Rationally, screening and optimisation studies at pilot scale from a biomanufacturing perspective would require more resources and intensive labour; it would be difficult if not impossible to alter the different variables in optimising productivity/quality, and it would not prove economically feasible. Therefore, from a biomanufacturing perspective, multiplexing NIRS provides the prospect of assessing the predictive ability of small scale models on large scale vessels, as developing models on large scale vessel will be constrained and this would also provide the opportunity to understand whether the model can account for the scale effect.
Conclusion
The technology has definitely matured enough to be implemented for the in situ monitoring of multiple vessels. It appears to be a superior technique in comparison to its competitors: mid-IR, UV-Vis and Raman, providing real-time information of the bioprocess on a routine basis efficiently and effectively.
The inherent advantages of this multiplex technology enables the user to obtain a much higher frequency of data in comparison to the conventional in situ technique, which implies that the process operator can rapidly monitor the progress of the process and in turn, optimised process control. From the perspective of the biopharmaceutical industry, this approach is beneficial as a number of reactors at different scales can be monitored simultaneously which will accelerate the process development stage and help meet project deadlines.
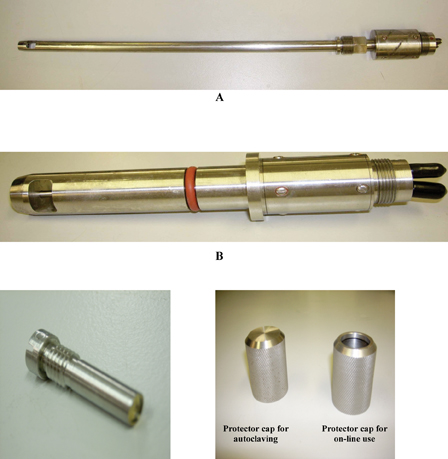
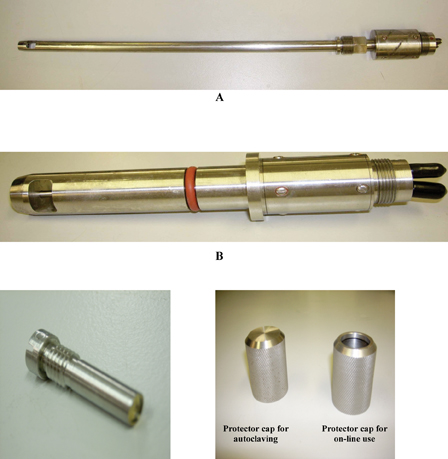
Figure 1: NIR accessories: A) 12mm probe B) 25mm probe C) detachable mirrors D) protective caps used in the in situ process monitoring
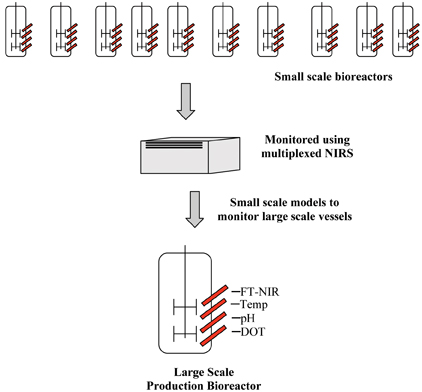
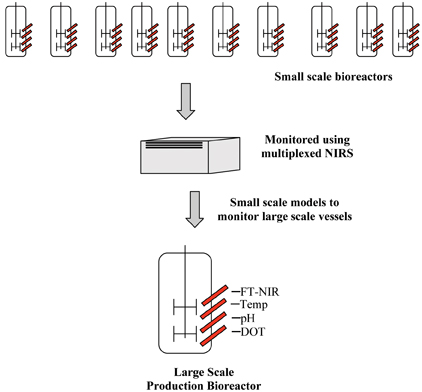
Figure 2: Pictorial representation of the small scale models developed using multiplexed NIRS to monitor large scale vessels
References:
- Bradshaw, T. Catalyzing UK’S bioprocessing sector. BioWorld Europe. 03, (2005).
- http://www.fda.gov/CDER/GUIDANCE/6419fnl.pdf
- Bozhurt, A. Rosen, A. Rosen, H. Onaral, B. A portable near infrared spectroscopy system for bedside monitoring of newborn brain. Biomed Eng Online. 4, 29, (2005).
- Arnold, A.S. Crowley, J. Woods, N. Harvey, L.M. McNeil, B. In situ near infrared spectroscopy to monitor key analytes in mammalian cell cultivation. Biotech. Bioeng. 84, 13-19, (2003).
- Roychoudhury, P. O’ Kennedy, R. McNeil, B. Harvey, L.M. Multiplexing fibre optic Near Infrared (NIR) spectroscopy as an emerging technology to monitor industrial bioprocesses. Anal. Chim. Acta. 590, 110-117 (2007).
Payal Roy-Choudhury
Payal completed her Bachelors’ degree in Biotechnology from Bangalore University in 2000. She then went on to complete a Master’s in Applied Genetics from Bangalore University in 2002. Payal was a recipient of the Gold Medal award for attaining 1st Rank in her Masters award, and was also awarded the Late Chikkalingappa & Gowramma Award, from Bangalore University, India.
As well as completing her final year PhD at The University of Strathclyde on the topic of “Infrared spectroscopic techniques to monitor industrial bioprocesses”, Payal has completed an industrial traineeship as part of final year PhD studies on “Multiplexing fibre optic near infrared spectroscopy (NIRS) to monitor animal cell culture processes” at GlaxoSmithKline, Beckenham, UK. In addition to publishing 4 papers on employing mid-end NIRS to monitor bioprocesses in the Journal ‘Analytica Chimica Acta’, Payal has presented posters at a number of prestigious conferences. Payal has recently received the Corporate Activities Program (CAP) Student Travel Grant from the American Society of Microbiology to present a poster at 107th ASM conference.