PAT for packaging: review of applications for expeditious, nondestructive quality testing
Posted: 15 April 2014 | | No comments yet
This article reviews some emerging applications of Process Analytical Technologies (PAT) for packaging quality testing. Specifically, four commercially-available packaging applications are explored in further detail: Raman spectroscopy for rapid material identification testing of polymeric packaging materials; vision-based elastic deformation for non-destructive blister integrity testing; X-ray monitoring for inline blister fill inspection; and thermal imaging for inline verification of bottle foil seal integrity. For each application, a brief review is provided stating technology capabilities and principles of measurement as well as sharing example(s), other relevant remarks covering some practical aspects and comments on considerations for building a business case for these technologies.
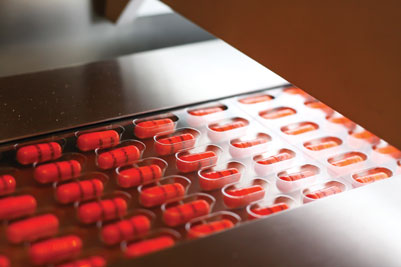
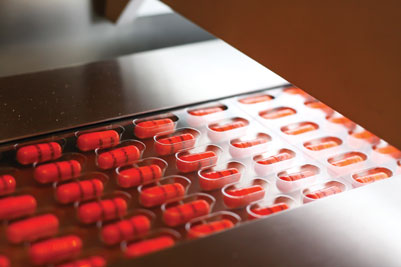
Raman for packaging materials identification testing The use of handheld Raman spectroscopy has quickly emerged as a standard for quick, non-destructive identification (ID) testing of materials for diverse reasons including specificity, through-liner scanning capabilities, portability, etc.1 The measurement principle of Raman spectroscopy relies on inelastic scattering of monochromatic radiation2. Basically, a sample of interest is exposed to a monochromatic wavelength source and the scattered light is analysed to obtain information on material properties.
For sites with both manufacturing and packaging operations, the use of this application for testing of polymeric packaging materials may be seen as a natural expansion of the technology, following successful use for rapid ID testing of manufacturing raw materials. However, from experiential learning, ID testing of packaging components / bottles can develop into a PAT application of its own merit, the reason being mainly related to both material volumes and the relatively extensive sample preparation required to perform conventional laboratory ID tests.
For example, with previous implementation of the portable Raman instrument for ID testing of high density polyethylene (HDPE) bottles at a Pfizer manufacturing facility, significant testing time reductions were accomplished compared to the conventional test, contributing to an overall leaner process. In addition, the application provided safety benefits as material cutting technologies were used during sample preparation to conduct the conventional ID test. Using Raman for non-destructive HDPE bottle scanning / testing requires no sample preparation, thus helping to lower potential laboratory safety risks.
From a practical standpoint, the capabilities and portability of commercially-available Raman instruments provide the flexibility to maximise use of the instruments. This inherent flexibility – coupled with the relative lower cost when compared to some of the bench top units – also helps ease project justification. From a business standpoint, there is specific published guidance to estimate potential savings from implementation of portable Raman instruments3. Previous implementation experience has shown payback periods of a year or less (depending on project scope) for investments relating to portable Raman ID testing applications.
Vision-based system for blister integrity testing
Blister integrity is a regulatory requirement to check for proper sealing and the absence of pinholes in blister packed pharmaceuticals. The blue dye test is currently the industry standard for testing blister integrity. This test involves the submersion of blister packs into a methylene blue dye solution for a period of time and then performing visual inspection of the individual pockets for dye ingress. Some of the inherent disadvantages with the blue dye test are that it is a destructive and thus potentially costly test (pharmaceutical product and packaging materials are destroyed during this test), sampling frequencies are low, and the test is laborious and time consuming. The blue-dye test also requires trained personnel to run the test and relies on subjective analysis of dye ingress into the cavities to identify blister leaks.
It is highly desirable to use an empirical technology solution that can eliminate some of the known disadvantages of the blue dye test whilst increasing limit-of-detection capabilities in terms of detectable pinhole size. One of these options is a vision-based system, which is capable of non-destructively assessing blister integrity for multiple sizes, shapes, cavity configurations and materials of construction. The test is relatively fast (about one minute per test cycle after method development), empirical, and provides pass / fail results eliminating any need for human-visual analysis of samples and associated subjectivity. These vision-based systems also allow for tool-less analysis of blister packs which allows rapid development and deployment of methods for almost all blister products manufactured at a site.
In terms of practical aspects, these systems are relatively simple to install as well as operate, and they are mostly intended as bench top units for off-line or at-line testing. The instrument applies cycles of both positive pressure and vacuum to a blister-holding chamber while an embedded camera captures images of the blisters at the different pressure / vacuum stages. It then utilises image analysis algorithms to compare images and identify subtle elastic deformations in individual blister cavities due to pressure changes. Sealed blister cavities will show deformation with pressure changes; and leaking blister cavities will show little or no deformation due to free passage of air (i.e. pressure equilibrium between the inside and outside of the leaking cavity).
Considering some of the financial statistics (return-on-investment, payback period, etc.), these systems can achieve favourable and appealing numbers, especially if matched for use with blister products exhibiting relatively high volume and / or high manufacturing costs per unit, when taking into account savings from the non-destructive nature of the test. In addition, due to the seemingly low complexity of the system hardware, and the fact that some systems do not require product-specific tooling, expected post-purchase cost of ownership is low.
X-ray for inline blister inspection
Inline systems using X-ray technology for blister inspection – mostly for empty blister cavities detection – have been commercially available for a while. However, given the capabilities this technology brings, and the reenergised industry efforts placed on reducing the costs of poor quality in support of leaner operations, it is worth considering these systems in greater depth. In general, the use of X-ray inspection in the pharmaceutical industry provides an alternate means of inspection for operations in which other inspection technologies such as vision based systems may not be suitable, for example, foil packaging.
In principle, X-rays have the ability to penetrate solids and ionise gases. The electromagnetic radiation of X-rays has a wavelength range of 3×1016 Hz to 3×1019 Hz (0.01 to 10 nanometres)4. As reference, they are shorter in wavelength than UV rays (and visible light) and longer than gamma rays. X-rays can penetrate objects to varying depths depending on intensity. Typical industrial systems utilise low-energy radiation to penetrate the samples of interest, and software analyses data enabling reject of out-of-specification blisters.
At the operational level, the system is relatively easy to set-up. Among the most important parameters are the configuration of the area of scrutiny for each blister cavity, and the selection of the ‘golden’ (reference) blister samples for initial set-up. The threshold limits and all X-ray data generated will be relative to these ‘golden’ blister samples. The defects that X-ray systems are able to detect typically include missing product in blister cavities, missing blister(s) in box packages (potentially eliminating the use of a check-weigher), partial and/or damaged product in blister cavities, and some types of damaged blisters.
From a business standpoint, adoption of this technology can help support cost avoidances, especially if any of the issues that the technology detects have a history of triggering customer complaints. In addition, adoption of the application supports elimination of off- / at-line testing for missing blister(s) in box packages and therefore cost improvements may be possible to calculate. This application also enables 100 per cent inline verification and automatic reject of defective / damaged packaged product, a level of quality assurance that, if required, would otherwise be considerably time and resource consuming. On the down side, depending on the product and the application of the X-ray technology, data may be required to demonstrate that there is no impact to a product’s efficacy and stability as a result of the application of X-ray radiation.
Thermal imaging for inline bottle foil seal integrity verification
The increasing availability and diversification in industrial use of thermal imaging technology, which was originally developed for defence applications (a typical example being night vision), has reached the pharmaceutical industry. Technology offerings are now commercially available, enabling first-ever inline inspection capabilities such as bottle foil seal integrity verification.
Warm bodies emit electromagnetic radiation due to thermal motion of their molecules5. In principle, thermal imaging cameras detect the radiation emitted by objects above absolute zero. Thermographs capture information on temperature variations given that the amount of radiation emitted by an object in the infrared spectrum increases with temperature. Wavelengths emitted by objects are able to penetrate most plastics, and can thus provide images of covered foil that are not possible with visible light-based imaging systems.
In the case of a bottle packaging / foil seal verification application, the thermal imaging camera is positioned after the induction sealing step. The system is able to capture patterns of temperature that are then analysed with image processing software. Pre-determined thresholds for thermal pattern variability allow detection of a range of defects associated to bottle foil seal integrity. Based on previous empirical experience, defects that can typically be detected using inline inspection with thermal imaging technology include:
- Bottles without caps
- Bottles with caps but without seal
- Bottles with skewed caps
- Bottles with high cap / unengaged cap
- Bottles with over-heated seals
- Bottles with under-heated seals
- Bottles with broken liner
From a practical standpoint, the system is relatively easy to adapt as an additional inline standalone unit to an existing bottle packaging line, assuming the additional required space is available. The full system incorporates a thermal imaging camera, a computer for data acquisition / image analysis / data logging, position sensors, and a reject module. Given that just recently this system became commercially available, it is worth noting some of the system benefits as demonstrated during a recent plant trial:
- No radiation is emitted by the system. The camera captures the infrared radiation emitted by the foil seal exiting the induction sealing machine; therefore, there is no risk of impacting product stability
- No interference with process speeds. In the case of our particular application, the system was capable of inline inspection at typical conveyor speeds
- Ability to collect thermal imaging data relating to foil seal integrity through the caps
- System software was developed targeting the pharmaceutical industry (21 CFR Part 11 Compliance)
- Aside from rejecting bottles with bad seals, the system is practically non-invasive – the camera captures thermal information as bottles pass through a conventional conveyor.
From a financial and investment standpoint, given the range of defects that the system can potentially capture, it is worth considering the type of historical defects, customer complaints, investigation efforts, and/or type of routine off-line inspection (as applicable). This would allow quantification of potential cost avoidances and/or cost improvements from purchase and installation of a thermal imaging system for inline verification of bottle foil seal integrity. Thermal imaging technology, in general, keeps evolving with considerable improvements relating to size reduction of equipment offerings, increased resolution, reliability, and software analysis capabilities; and greater price accessibility.
Concluding remarks
This article is aimed at providing an overview of some of the packaging PAT applications that are emerging and enabling expeditious, non-destructive quality testing. Four applications were reviewed that included: 1) Handheld Raman for packaging raw material identification testing, 2) Vision-based system for blister integrity testing, 3) X-ray monitoring for inline blister inspection, and 4) Thermal imaging for inline bottle foil seal integrity verification. The technology behind each PAT application was briefly explained, an example provided through visuals, and some of the practical and financial considerations mentioned.
In general, we have seen progress in the number and type of PAT applications that can support packaging operations. This shows not only the diversification of PAT to expand the impact of technologies across the different pharmaceutical operations, but also the continued commitment on innovation by vendors and manufacturers in search of alternatives to reduce the costs of quality while maintaining or increasing the levels of quality assurance.
While cost reduction and economic pressures continue to play a key role in the pharmaceutical industry, we cannot deny the fact that technology with a purpose – that is, to improve quality, reduce rejected product, lower manufacturing costs, etc. – can help create a competitive advantage. Once suitable technological applications are identified and business cases are clearly developed, the sum of investment decisions is likely to play a role in cost-effectiveness and thus, long-term sustainability of existing manufacturing operations.
References
- An Implementation Perspective on Handheld Raman Spectrometers for the Verification of Material Identity. B. Diehl, C.S. Chen, B. Grout, J. Hernandez, S. O’Neill, C. McSweeney, J. Montenegro- Alvarado and M. Smith (2012). European Pharmaceutical Review, Non-destructive Materials Identification Supplement, Volume 17, Issue 5, 2012. Electronically retrieved on October 2013 from http://www.europeanpharmaceuticalreview.com/wp-content/uploads/Raman-Supplement-2012.pdf
- An Introduction to Raman Spectroscopy: Introduction and Basic Principles. J. Javier (2005). Electronically retrieved on October 2013 from spectroscopyNOW.com at http://www.spectroscopynow.com/details/education/sepspec1882education/An-Introduction-to-Raman-Spectroscopy-Introduction-and-Basic-Principles.html?tzcheck=1
- Portable Raman for Raw Material QC: What’s the ROI? E. L. Diz & R. Thomas (2013). Electronically retrieved on October 2013 from PharmaManufacturing.com at http://www.pharmamanufacturing.com/articles/2013/006/?start=1
- Regions of the Electromagnetic Spectrum. Electronically retrieved on November 2013 from NASA at http://imagine.gsfc.nasa.gov/docs/science/know_l1/spectrum_chart.html
- Summary of Black-Body Radiation Theory and Observations. J. Mallinckrodt (2010). Electronically retrieved on November 2013 from California State Polytechnic University, Pomona at http://www.csupomona.edu/~ajm/classes/phy235/blackbody.pdf
Biography
José Montenegro-Alvarado is Manager of PAT Projects based at Pfizer in Vega Baja, Puerto Rico. He is currently responsible for technical support and facilitates implementation of PAT at Pfizer’s Pharma Operations / Solid Oral Dosage, Consumer Health, and Local Markets operating units’ sites in Puerto Rico, Australia, and Argentina. José has 15 years of pharmaceutical operations experience covering process research, capital project management, technical support, and PAT installations. José earned a BS (honours) and MS degrees in Chemical Engineering (University of Puerto Rico at Mayaguez), and holds a Professional Engineer (PE) license.
Bradley (Brad) Diehl is Manager of PAT Projects at Pfizer in Peapack, New Jersey. His primary role is to engage and facilitate appropriate implementation of PAT at Pfizer manufacturing sites. Brad has 29 years of process analytics experience including applications for products and processes in consumer healthcare, human health, animal health, and petrochemical. Brad earned a BA in Chemistry/minor in Biology (Shippensburg University, PA), MBA (University of Tulsa, OK), and MS in Quality Assurance/Regulatory Affairs (Temple University, PA).
Jean-Maxime Guay is Scientist of PAT projects at Pfizer located in Montreal, Canada. He joined Pfizer’s Process Analytical Sciences Group (PASG) in 2010 as a master’s degree student in chemical engineering (University of Sherbrooke, Canada). Jean-Maxime previously earned BA degrees in bioinformatics and biotechnological engineering. He is currently responsible for the technical support and implementation of Rapid Analytics in Canada/US manufacturing and product development sites.
Steve Hammond is Senior Director / Team Leader of Pfizer’s Process Analytical Sciences Group (PASG) based in Peapack, New Jersey. Steve is a Graduate of the Royal Society of Chemistry in the United Kingdom. He held analytical chemistry roles within Anglo American and Unilever, before joining Pfizer in 1979. Over the last 30+ years, he has fulfilled several analytical roles within Pfizer, specialising in the field of Process Analytical Technology and leading its application to improve the performance of Pfizer manufacturing operations worldwide.
Hiwot Isaac is Manager of PAT Projects based in Peapack, New Jersey. She is currently responsible for technical support and implementation of PAT at Pfizer’s Local Markets operating unit sites in North Africa, Middle East and Europe. Hiwot earned a Bachelor of Pharmacy (Haile Selassie I University, Addis Ababa Ethiopia) and MS degree in Pharmaceutical Chemistry (School of Pharmacy University of IFE, Nigeria). She has 25 years of experience in the pharmaceutical industry and has worked in R&D and technical services roles. Her credentials include: New Jersey Pharmaceutical Quality Control certificate (NJ Pharm), Pharmacy License, University of State of New York Education Department, Research Fellow and University of Uppsala, Sweden.
Ben Lyons is Senior Scientist of PAT Projects based in Peapack, New Jersey. Ben joined PASG in 2003 and has worked extensively on PAT development projects. He currently supports deployment of Process Analytics to Solids site manufacturing. Ben earned a degree in Forensic and Analytical Chemistry (University of Strathclyde at Glasgow, Scotland).
Conor McSweeney is Senior Manager of PAT Projects at Pfizer in Cork, Ireland. He is responsible for supporting the implementation of PAT at a number of the Solid Oral Dose sites in Pfizer. He worked in the Loughbeg API plant in Ireland as an Analytical Chemistry Specialist from 1998 -2001 and then moved to the Loughbeg Drug product plant and took up the role as Chemistry laboratory Co-ordinator. He then started working with the Process Analytical Sciences Group in 2006 as a Senior Scientist, was promoted to Manager-PAT project in 2008 and to Senior Manager-PAT Projects in 2013. Conor earned an Honours Chemistry Degree and PhD in Analytical Chemistry from University College Cork.
Seamus O’Neill is Director / Team Leader located in Cork, Ireland. He leads a team that supports the implementation of PAT across all sites in Pfizer’s Pharma Operations and Speciality Bios operating units. Seamus has held roles of increasing responsibility in analytical chemistry within Pfizer and previously in analytical development at Nycomed Amersham, Warner Lambert, Clonmel Healthcare, and GlaxoSmithKline. Seamus’s academic studies in Analytical Chemistry were completed at the Cork Institute of Technology in Cork, Ireland.
Jean-Sébastien Simard is Senior Manager / Team Leader located in Montreal, Canada. He has a Bachelor and a Master degree in Chemical Engineering from Université de Sherbrooke, Québec, Canada; and is currently pursuing a MBA degree at Université Laval, Québec. He started in 2002 with Wyeth Canada, where he worked as a Product and Process Development Scientist, and then became responsible for the PAT Development Group of the Technical Services in Montréal. Jean-Sébastien now leads a team that supports the implementation of PAT across all sites in Pfizer’s Consumer Health and Local Markets operating units. He is also the Industrial Responsible of the Université de Sherbrooke/Pfizer Industrial Research Chair on PAT in Pharmaceutical Engineering.
Joep Timmermans is Director / Team Leader based in Peapack, New Jersey. Joep leads the Platform Technology Development team within the PASG. Joep has been at Pfizer for over 10 years. Prior to that, he worked at Merck and Co for nine years in both PAT as well as site Technical Services. He is co-chair of the ISPE PAT Community of Practice Steering Committee as well as committee member of the Pharmaceutical Process Analytics Roundtable. He also contributes to other initiatives such as ASTM Committee E55, PhRMA, and PQRI. Joep earned a Doctoraal degree in Physics (University of Leiden) and a PhD in Physical Chemistry (Princeton University).