Real-time biological particle counting in environmental monitoring
Posted: 20 April 2015 | Tim Sandle
The methods for monitoring air in cleanroom environments: viable counting techniques (settle plates and biological air samplers) and particle counters, are long established technologies and have been widely used in pharmaceutical manufacturing environments for decades. Although innovations have taken place with both particle counters and biological air samplers, primarily in relation to the size of the instruments and in reducing the time taken to collect a fixed volume of air, the essential technology has remained unchanged. However, recently, some new technologies for biological air sampling have been developed (bio-air systems), which will be discussed in this article.
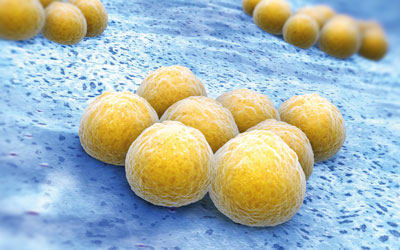
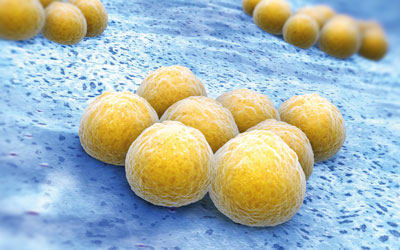
The new technologies to be developed are optical spectroscopy counting instruments designed to simultaneously detect the number and size of particles from a volume of air (and thus enable ‘real-time’ microbiological assessment.) Such systems operate in a similar way to conventional particle counters, which measure inert particles as part of the GMP assessment of cleanrooms (and comply with such cleanroom standards as ISO 14644). Here the instruments count airborne particles by measuring light scattering. The difference with the spectrophotometric counters over traditional counters is that the instruments are capable of determining if the detected particles are biological or inert. Thus the key innovation is that such devices can differentiate between non-viable particles and biological material, which may indicate if microorganisms are present in the sample of air.
The implementation of such technologies is in keeping with the industry and regulatory drive, particularly from the US Food and Drug Administration, for rapid methods and for pharmaceutical manufacturers to utilise the concept of process analytical technology (PAT). PAT is a set of mechanisms to design, analyse, and control pharmaceutical manufacturing processes through the measurement of critical process parameters. With the PAT philosophy the ideal is to make such assessments in ‘real-time’, so that quality issues can be addressed during batch manufacture.
In terms of advantages to microbiologists and cleanroom managers, the new generation of optical counting instruments will not only provide a real time assessment of the probable numbers of microorganisms in the air but will also detect microorganisms that cannot be recovered using conventional culture-based microbiological methods. The reason why conventional cultural-based environmental monitoring methods are limited is multifaceted. One reason is because only small samples sizes are taken. A second reason is that there is no universal culture medium or set of incubation conditions which will grow all microorganisms2. Moreover there are many ‘viable but non-culturable microorganisms’3 found in cleanrooms. The term ‘viable but non-culturable microorganisms’ describes bacterial cells which, whilst maintaining certain features of viable cells (measurable metabolic activity), will not culture on agar4. Theoretically real-time technologies will be able to detect these non-culturable microorganisms. A further factor is that drawing a correlation between non-viable and viable particles from a given volume of air is problematic5.
Bio-air systems and their application
There are different bio-air systems on the market. They tend to function in similar ways, although there are commercial differences. They are relatively immature technologies, having been commercially available for the past five years, and the application of the measurement of biologic particle concentrations using a fluorescent particle is relatively novel6.
The different systems are similar in terms of their design and biophotometry technology6. In terms of design, they are instruments housed in stainless boxes and the air is drawn in through an isokinetic probe powered by an air pump. In terms of the technology, the core part of the systems consists of an optical laser. When a particle passes through the laser it intersects the laser. A particle size detector is then used to measure individual particle sizes (similar to established particle counters, based on the Lorenz–Mie solution which formulates the scattering of electromagnetic radiation by a particle. Here the amount of light scattered can be used to calculate the size of the particle)7. In addition, there is a parallel optical assembly (photodetector) to detect an ultra violet laser-induced fluorescence signal, which is triggered by certain metabolites inside microorganisms. The collected data is analysed by computer software which is able to differentiate between inert particles and microorganisms.
The viable counting technology of the instruments functions on the basis that microorganisms contain metabolites. Certain metabolites are ‘excited’ by a specific wavelength of light. The metabolites looked for are the co-enzyme nicotinamide adenine dinucleotide (NADH), dipicolinic acid and the micronutrient riboflavin (vitamin B2). These metabolites are found within all cells. The excitation of these metabolites, using ultra violet photon energy (at a wavelength of 405nm), causes the emission of auto-fluorescence light, which the instrument then detects. Fluorescence detection is well-suited for detection of microbial contamination as it has a high sensitivity and requires only a short collection time. The design of the instruments allows them to scan large volumes and to express results per cubic metre of air. The systems use different preparatory units of measurement, although they are similar in that each unit of measurement is said to be equivalent to one colony forming unit (as would be recorded from a conventional culture based environmental monitoring method)8.
The instruments are being marketed for different applications. One such use is for aseptic filling operations with the idea that those involved with sterile filling will be able to detect not only particles, but also biological activity. This would allow cleanroom operators to stop the process if microorganisms are suspected to be present in the air. Action can then be taken to address the contamination or to segregate a portion of the batch. The ability to instantaneously detect microorganisms is a significant advance as conventional methods require several days to culture bacteria and fungi. In such scenarios, the systems can be used for continuous or episodic monitoring.
Another use is using the instrumentation to set a benchmark and then taking measurements in the event of an incident to determine if a suitable recovery has occurred, for example, following an air handling system failure. A further application is in collecting data about cleanrooms, such as assessing the maximum number of personnel allowed within a cleanroom with a given air-exchange rate9. Another advantage is the ability to trend and the possibility of correlating airborne particles with microorganisms. Indeed the technology may possibly be able to show that a rise in concentration of ≥0.5µm particles above a certain threshold will provide an early warning of a rise in microorganisms.
Points for consideration
There are, however, some important points to consider with regard to the future application of the technology.
The technologies require considerable validation and method development before they can be used for batch release. As with particle counters, the validation relies upon the instrument being able to calculate and recover known particles. Criteria such as precision, robustness and limit of detection should each be assessed. The systems must also be able to distinguish between inert particles and viable particles and not react to any other substance which might trigger fluorescence. One study showed a vulnerability, in terms of reacting to disinfectant sprays and clothing fibres10.
Moreover, it is important and indeed essential with viable microorganisms that the limit of detection and limit of quantification is assessed, since regulatory standards require monitoring systems to be able to detect one colony forming unit in a cubic metre of air.
A more difficult aspect is the method development. Since the level of microorganisms present in cleanroom air but not detected by conventional methods cannot be quantified, direct parallels cannot be made. Furthermore, suppose within a Grade A / ISO class 5 clean zone there is one microorganism and a real-time counting device is run alongside a settle plate. The single organism may be captured by the real-time device or it may fall onto the settle plate. It cannot be detected in both and in such a situation there is no direct comparability. Comparative analysis can be undertaken using a bioaerosol chamber and a single, mixed population of microorganisms. However, the problem of assessing the instrument in situ remains.
The next important point is that real-time technology is a destructive method. It will indicate that a microorganism is present but it will not determine the species. Although some would argue that with sterile manufacturing the species is less relevant because any microorganism presents a risk, knowing the species allows the origin of the contamination to be assessed (a Staphylococus spp., for example, indicates an association with human skin) and for comparisons to be made with different environments (if the same microorganism is recovered at Grade A/ISO class 5 as is recovered at Grade B/ISO class 7, then they may be a connection). This means that conventional methods would be required to be run alongside real-time technology.
A final consideration rests on the logistics of using real-time. If a suspected microbial event occurs, what is the appropriate response? Is it to suspend filling? What happens if further events occur on re-start: is this batch rejection? If no further events are detected is a portion of a batch to be rejected? Or is there, as with conventional methods, no direct link between detection of contamination and product risk? Furthermore, what is the appropriate response if conventional methods detect microorganisms and real-time methods do not, or vice versa? Finally, what does this mean for established alert and action levels? These questions pose dilemmas for the pharmaceutical manufacturer to resolve.
If these points may appear to dampen the potential of such technologies, they are not intended to do so. Nonetheless, these are questions which require unravelling as real-time technologies become established. There is little doubt, not least given the considerable development costs, that these systems will become accepted methodologies.
Summary
Real-time instantaneous monitoring systems, capable of detecting and differentiating between non-viable and viable particles in the air, are progressing and several systems are being trialled in pharmaceutical organisations. Such systems offer advantages in providing more detailed information about the status of clean zones and can potentially act as useful investigational tools. In the longer-term the technology has the potential to replace conventional methods entirely once some of the points posed in this paper are resolved. The most significant of these is the speciation question: how can microbiologists reliably determine the species of microorganism and relate this to a possible source or location?
These issues notwithstanding at some future point real-time monitoring systems will probably become part of the accepted methods used for assessing non-viable and viable particulates within pharmaceutical grade cleanrooms.
References
- FDA (2004). Guidance for industry: PAT – A framework for innovative pharmaceutical development, manufacturing and quality assurance”. Food and Drug Administration, Rockville: MD, USA.
- Sandle, T. (2014) Examination of the Order of Incubation for the Recovery of Bacteria and Fungi from Pharmaceutical Cleanrooms, International Journal of Pharmaceutical Compounding, 18 (3): 242 – 247
- Weichart, D. (1999). “Stability and survival of VBNC cells – conceptual and practical implications”. In Bell, C.B-G. (Ed.), Proceedings of the 8th Symposium on Microbial Ecology, Atlantic Canada Society for Microbial Ecology, Halifax: Canada.
- Tidswell, E. (2011). “Sterility”. In Saghee, M. Sandle, T. and Tidswell, E. Microbiology and Sterility Assurance in Pharmaceuticals and Medical Devices, Business Horizons, New Delhi: India.
- Raval JS, Koch E, Donnenberg AD. (2012) Real-time monitoring of non-viable airborne particles correlates with airborne colonies and represents an acceptable surrogate for daily assessment of cell-processing cleanroom performance, Cytotherapy. 14(9):1144-50
- Dai C, Zhang Y, Ma X, Yin M, Zheng H, Gu X, Xie S, Jia H, Zhang L, Zhang W (2015) Real-time measurements of airborne biologic particles using fluorescent particle counter to evaluate microbial contamination: Results of a comparative study in an operating theatre, Am J Infect Control. 43(1):78-81
- Miller, M. J.; Lindsay, H.; Valverde-Ventura, R.; O’Conner, M. J. Evaluation of the BioVigilant IMD-A, a novel optical spectroscopy technology for the continuous and real-time environmental monitoring of viable and nonviable particles. Part I. Review of the technology and comparative studies with conventional methods. PDA J. Pharm. Sci. Technol. 2009, Vol. 63, No. 3, pp244 –257
- Sandle, T.; Leavy, C.; Jindal, H.; Rhodes, R. Application of Rapid Microbiological Methods for the Risk Assessment of Controlled Biopharmaceutical Environments. Journal of Applied Microbiology. 116(6): 1495-1505
- Sandle, T., Leavy, C. and Rhodes, R. (2015) Assessing airborne contamination using a novel rapid microbiological method, European Journal of Parenteral & Pharmaceutical Sciences, 19(4): 131-142
- Eaton, T.; Wardle, C.; Whyte, W. Use of a Real-Time Microbial Air Sampler for Operational Cleanroom Monitoring. PDA Journal of Pharmaceutical Science and Technology. 68(2): 172-184
Biography
Dr Sandle’s primary role is Head of Microbiology at Bio Products Laboratory, a sterile products manufacturer. In addition, he is a tutor with the School of Pharmacy and Pharmaceutical Sciences, University of Manchester for the university’s pharmaceutical microbiology MSc course and a longstanding committee member of the pharmaceutical microbiology society Pharmig.
Issue
Related topics
Airborne particle counting, Environmental Monitoring, Process Analytical Technologies (PAT)