PAT Series: Applicability of process analytical tools to bioprocessing trends
Posted: 3 July 2015 |
The past decade has been a period of unparalleled change and development in the fermentation industry. As the nature of this industry evolves, and in particular, with the increasing prominence of the new biopharmaceuticals (therapeutic proteins, diagnostic enzymes and monoclonal antibodies) the need for effective bioprocess monitoring grows in importance1.
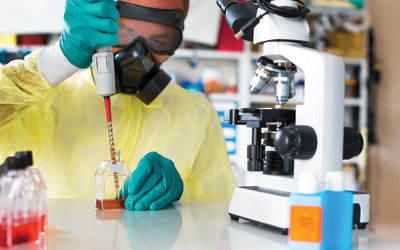
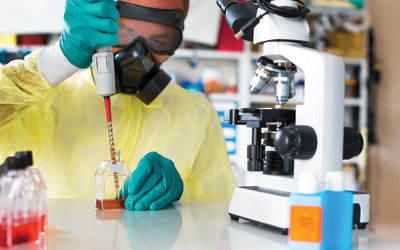
In order to deliver the ‘revolution in clinical medicine’ promised by this new range of therapeutic agents, there is a pressing need to develop effective bioprocess monitoring techniques and technologies which can deliver robust, reproducible, stable manufacturing processes, as well as high quality data, not just on product levels but also relating to product authenticity and purity. So what is wrong with the current manufacturing practice in the biomanufacturing industry? Today, most fermentation processes suffer from poorly understood process interactions, empirical risk assessment and process locked in by validation; therefore, the existing procedural approach has helped foster ‘a climate of fear’ of innovation itself.
In its process analytical technology (PAT) initiative, the US Food Drug Administration (FDA) has outlined a system for designing, analysing and controlling manufacturing through timely measurements of critical quality and performance attributes of raw and in-process materials, with the goal of ensuring final product quality2. This has led many pharma companies to re-evaluate their requirements for bioprocess analysis.
The PAT initiative encourages the development of improved process monitoring technology in the manufacturing environment. Therefore, the characteristics of the ‘ideal’ bioprocess monitoring technology are usually accepted as including the following: rapid, nondestructive, generating multi-analyte data, operable in (near) real time, capable of automation, robust, sensitive, and provision of data that is amenable to integration with information from other sensor types.
PAT tools
Few technologies seem capable of delivering all characteristics of the ‘ideal’, however; perhaps vibrational spectroscopy is the technology that most closely approaches this level. Near-infrared (NIR) and raman are now among the most common, prevalent sensors in real-time biopharmaceutical monitoring.
In comparison to NIR and raman spectroscopy techniques, the corresponding mid infrared (MIR) spectrum of a sample exhibits a high degree of spectral resolution; as a result, peaks can effectively be assigned to specific chemical entities or individual product constituents. Nonetheless, the application of the MIR to quantify multiple components in aqueous solutions has not been common for a number of reasons, such as very pronounced water absorptions, labourious sample preparation involving Nujol mulls or potassium bromide (KBr) disks, and instrumentation that was designed more for laboratory analysis than for real-time measurements.
This compares with both NIR and raman spectroscopic techniques, which offer simultaneous, real-time multi-analyte information within minutes (fewer than two usually), and since information about all analytes in the fermentation fluid is usually included in the spectra, a ‘metabolic snapshot’ is potentially achievable using these technologies. These techniques are robust, scalable, and capture tremendous quantities of process data. However, they both require sophisticated chemometric techniques to correlate spectral information with that of analyte-specific information.
NIR evolution
The use of NIR has evolved, initially from raw materials testing, and then to final product quality control, and most recently to process monitoring. Advances in computing and chemometrics have contributed to this growth, together with the realisation that NIR is suitable for microbial, fungal, yeast, and mammalian cell cultures (although not equally). NIR’s appeal stems from its advantages over traditional in- or at-line techniques: flexibility, simplicity of operation, rapidity of measurement, multiplicity of analysis, and the potential to predict chemical and physical parameters from a single spectrum.
NIR spectra are collected over the entire spectral range, from 800 to 2,200 nm, and provide multianalyte readings simultaneously in real time including cell counts, metabolite and nutrient uptake rates, and product levels. In addition, NIR works especially well in cell culture (vs. fermentations) because the medium is clear and cell density, gassing, and agitation are low compared with microbial cultures.
Yet despite fulfilling nearly all objectives of the FDA’s PAT initiative, the industry’s adoption of NIR has been less than inspiring. I previously worked at AstraZeneca, for instance, and as such I have experimented with NIR at the bench scale, but I did not find an opportunity to explore it at pilot scale. I believe that the potential of spectroscopic techniques is not used as extensively in process monitoring as it should be.
NIR has numerous advantages for PAT programmes, particularly with respect to critical to- quality factors. NIR provides rapid, real-time, non-invasive, cost-effective monitoring with no sampling or sample preparation. Furthermore, it may be multiplexed with respect to both parameters and bioreactors. It is also scalable and transfers well. This is really important, as in its life cycle a typical biopharm product will transfer across scales and relocate geographically. Analytical models that are robust in the face of such changes are potentially applicable throughout the product’s life cycle.
NIR is not perfect. It is considered a secondary technique, meaning that it does not directly measure analytes through intimate contact with a sensor. Users must expend significant effort into modelling, and the stability of fibre-optic probes it employs has been questioned. For example, after repeated sterilisation cycles, the NIR probe might undergo signal saturation or show drifting in spectral signals. The fibre optics available today are generally made of silica or quartz and are quite fragile. Microfractures may develop over time, leading to complete loss in transmission.
Lately, raman spectroscopy has been receiving a lot of recognition in monitoring of cell culture processes. Basically, both raman and NIR are complementary techniques. IR is based on absorption, whereas raman gives rise to scattering. The spectra in fact appear almost as mirror images. For both methods the location of the signal indicates what is being measured, and the height tells how much. Moreover, both the techniques require chemometrics for relating spectral bands from compounds of interest to the test system.
However, making predictions from NIR and raman can be only as good as the reference assay method allows. What raman micro spectroscopy does is combine high quality visible images with high laterally resolved molecular information. However, questions about the appropriate equipment, i.e., choice of excitation wavelength, do arise when samples tend to fluoresce or heat. Yet the technique has improved over the years. Instruments are smaller and much less expensive; advances in electronic and optical components have greatly improved raman signals and expanded the technique’s suitability for monitoring bio- and other process industries.
Having discussed the two technologies, the next topic for consideration would be the mathematical modelling, involved in correlating the spectral information with reference assay. Analytical modelling requires the use of sophisticated mathematical treatments in correlating the spectral data with the reference data for the respective analytes. For complex matrices as those observed in fermentation fluids, partial least square (PLS) and principal component analysis (PCA) are the most commonly used techniques. There are a number of commercially available software packages which can be used to correlate the spectral information with the reference data such as: Vision, SIMCA, OPUS, Unscrambler and Matlab. Multivariate data analysis of the spectral and process information demonstrates a great deal of potential in bioprocess understanding and optimisation.
Despite the potential with spectroscopic techniques like raman and NIR, there still exist barriers to the adoption of the PAT technology. These include: investment of time, personnel, and equipment, particularly for one-off projects; validation and modelling; and the high level of user familiarity with the theory, mathematics, and instrumentation that is required. Nonetheless, the benefits of the technology in terms of attaining real-time process information outweigh the aforementioned limitations.
Industrial fermentation processes
Although all modes of bioprocessing, batch, fed-batch and continuous processes are currently used, most industrial fermentation processes are fed-batch. The typical fed-batch bioprocess usually involves very close control of one or more nutrients at very low (limiting) levels. This is also characteristic of chemostat continuous culture systems. This is an area where online tools such as raman and NIR may provide much more useful information as well as help give optimised control.
The recent industry trends show a shift towards ‘continuous biomanufacturing’. Continuous manufacturing is the method of prolonging the exponential phase of an organism whilst maintaining an environment that has less fluctuation in nutrients, cell number or biomass, also known as ‘steady state’. Continuous upstream bioprocessing involves retaining production cells at a fixed volume and at a fixed concentration on a continuous basis. However, this is not something new, first being used in 1980’s for classic fused cell hybridoma culture and now replaced by recombinant antibody manufacturing methods.
In a typical perfusion, the process vessel is inoculated and fed over time and the medium is removed from the vessel. To prevent the loss of cells, a perfusion device is placed on the medium take off. The duration of the process is, typically, from one to three months. It is important to take into account product quality and consistency, especially for long fermentation runs, since the product should be the same at the end of the process as it was at the beginning. Genzyme and Bayer are the leading industry adopters for perfusion processes currently, however, it is likely that more companies will turn to perfusion in the future.
Continuous manufacturing is ideal for products that raise quality concerns, since the bioreactor is continuously harvested and the labile products can be processed quickly. The operating conditions of the bioreactor are constant and can be precisely controlled and this may be critical for some products. Advantages of a perfusion process are the high volumetric productivity from the bioreactor as compared to fed-batch, the far higher peak cell densities that can be achieved and the ability to operate the bioreactor continuously for extended periods of time at these high cell densities. Above all, with a perfusion process, it enables quality to be built inherently into the process, leading to lower costs, increased productivity and superior quality.
In recent years, the FDA has delivered presentations highlighting the benefits of continuous manufacturing and describing how issues such as how to define lots have been resolved3. Therefore, it is clear there are no major regulatory hurdles for implementing continuous manufacturing. The FDA supports the implementation of continuous manufacturing using science- and risk-based approaches, since steady state operation offers the key advantages of consistent quality and reduced heterogeneity.
Most of all, combining a perfusion process with online monitoring PAT tools could provide much greater benefits to bioprocessing, in attaining real-time information, in turn, leading to effective process monitoring and thereby providing optimised control (Figure 1).
Conclusion
As the value of the biopharmaceutical market rises, and the economic pressures on products increase, many companies are seeking to introduce their biomanufacturing processes to PAT. At present, raman- and NIR-based solutions are among the leading candidates for generating improved process understanding in these complex processes. The future for PAT tools looks bright: it offers a quality by design approach in the context of the increasing industry-wide trend towards perfusion processes. And with the regulatory barriers to continuous manufacturing now being resolved, PAT can only continue to come on leaps and bounds.
References
- Roychoudhury, P. O’ Kennedy, R. McNeil, B. Harvey, L.M. Multiplexing fibre optic Near Infrared (NIR) spectroscopy as an emerging technology to monitor industrial bioprocesses. Chim. Acta. 590, 110- 117 (2007)
- http://www.fda.gov/CDER/GUIDANCE/6419fnl.pdf
- http://www.fda.gov/downloads/AboutFDA/CentersOffices/OfficeofMedicalProductsandTobacco/CDER/UCM341197.pdf
Biography
Payal Roychoudhury is a former employee of AstraZeneca (AZ) where she worked as Senior Cell culture Scientist at R&D Mölndal, Sweden. In 2009, Payal joined AZ and has extensive experience with near infrared spectroscopy (NIR) and bioprocessing. She has worked with microbial, mammalian, insect cells and Pichia cultures.
She currently works for ThermoFisher Scientific as Field Application Specialist, supporting Pharmaanalytics and Gibco customers both in UK/ Ireland. Prior to that, she worked as Lead Scientist for Lonza Biologics, Slough, supporting projects at the cell culture department. Payal completed her PhD on ‘Infrared spectroscopy to monitor industrial bioprocesses’ from the University of Strathclyde, Glasgow, UK. She worked as a post-doctoral research fellow at University of Strathclyde on the Department of Trade Industry (DTI) project with GlaxoSmithKline as industrial partner. In addition, she also worked as an NIR application scientist providing technical expertise to Bruker Optics customers (Coventry, U.K).