Cancer-secreted microRNAs: Travelers, Messengers, and Blood-borne Biomarkers
Posted: 22 February 2010 | Dr John Rossi, Professor and Chair, Division of Molecular Biology and Dean, Irell and Manella Graduate School of Biological Sciences, Beckman Research Institute of the City of Hope and Emily Wang, Assistant Professor, Deptartment of Cancer Biology/Division of Tumour Cell Biology, Beckman Research Institute of City of Hope
Since the first discovery of microRNA (miRNA) from C. elegans in 19931 studies of this new class of regulatory small RNA have grown rapidly and entered a new era, where they now serve as potential biomarkers and therapeutic targets in human diseases, such as cancers. Recent studies indicating that miRNAs are aberrantly expressed in cancer, are secreted by cancer cells, and are stably present in blood open a new avenue for studying cancer at the extra/intercellular level, where miRNAs serve as important cancer-released messages.
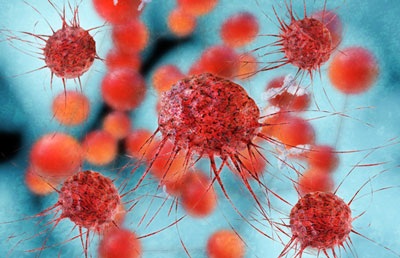
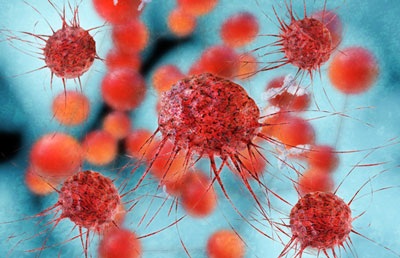
Since the first discovery of microRNA (miRNA) from C. elegans in 19931 studies of this new class of regulatory small RNA have grown rapidly and entered a new era, where they now serve as potential biomarkers and therapeutic targets in human diseases, such as cancers. Recent studies indicating that miRNAs are aberrantly expressed in cancer, are secreted by cancer cells, and are stably present in blood open a new avenue for studying cancer at the extra/intercellular level, where miRNAs serve as important cancer-released messages.
MiRNA dysregulation is related to human cancers
MiRNAs are naturally-occurring non-coding small RNA molecules of 21-24 nucleotides that most often form partially complementary base pairs within the 3’UTRs of mRNAs encoding proteins. Consistent with their regulatory function, miRNAs are crucial for development, differentiation, cell proliferation and apoptosis. In animals, each miRNA can downregulate protein expression from hundreds of target mRNAs via translational inhibition and destabilisation of mRNAs. Therefore, despite the small number of miRNA genes, which comprise less than a few percent of the genes in the human genome, miRNAs provide an efficient mechanism to exert genome-wide regulation, and simultaneously affect several cellular pathways. Most miRNAs are encoded within intergenic regions or the introns of protein-coding genes. The biogenesis of miRNAs is tightly controlled at both transcriptional and post-transcriptional levels, and involves processing of their hairpin structures from primary transcripts by RNAse family member enzymes Drosha and Dicer. Dysregulation of miRNAs are linked to various human diseases, especially cancers. Cancer-specific miRNAs have been identified in chronic lymphocytic leukaemia, glioblastoma, and cancers of the breast, lung, colon, thyroid, liver and pancreas. Additionally, they have shown promise as tissue-based markers for cancer classification and prognosis2,3. Certain miRNAs can function as tumour suppressors (e.g., miR-15a and miR-16-1) or oncogenes (e.g., miR-155 and the miR-17-92 cluster), and are involved in the initiation and progression of cancer2. Consistent with their relevance in cancer, >50% of miRNA genes are located at fragile sites in chromosomes and within regions of deletion or amplification that are genetically altered in human cancers4. A recent study demonstrated that viral vector-delivered miR-26a, an miRNA with reduced expression in liver cancer, inhibited cancer cell proliferation and disease progression without toxicity in a Myc-induced mouse model of hepatocarcinoma, suggesting that modifying miRNA expression may serve as a promising new approach in cancer treatment5,6.
About 800 miRNAs have been identified so far in humans, with a predicted total number of approximately 1,000. Microarray-based miRNA profiling studies have defined some miRNAs as predictive markers in different types of cancers. Compared to the large number of mRNA genes (~30,000 mRNAs per cell), the small number of miRNAs allows large-scale evaluation in patients with higher efficiency and lower cost. Because of their small size, miRNAs are relatively more stable in formalin-fixed, paraffin-embedded samples, and are therefore an ideal new tool for diagnosis. In a screening study using normal and neoplastic breast tissue samples, 29 miRNAs were differentially expressed in cancerous versus normal tissue3. Among these, miR-21 and miR-155 were most significantly upregulated in breast cancer tissue, whereas miR-125b and miR-145 were most potently downregulated. A set of 15 predictive miRNAs exhibits the power to differentiate normal versus breast cancer tissue. Moreover, expression of certain miRNAs is correlated with specific bio-pathologic features in breast cancer, such as estrogen and progesterone receptor expression, tumour stage, vascular invasion, or proliferation index3. Another study found that some miRNAs, including miR-200c-141, miR-200b-200a-429, and miR-183-96-182, are downregulated in cancer stem cells, indicating that these miRNA clusters may play a role in inhibiting clonal expansion and their restoration presents a potential therapeutic approach for treating cancer relapse from stem cell activation. Target mRNAs affected by these miRNAs encode proteins with roles in self-renewal (BM11), tumour formation (MYB, FOXO1A, IGFBP1, SOX2), and other crucial functions of tumour and embryogenic development7.
A body of previous studies used gene expression (mRNA/cDNA) arrays to profile tumours at the molecular level. As miRNAs are new players on the scene as markers for cancer diagnosis and prognosis, future integrated analyses of miRNA profiling and gene expression array profiling from cancer tissues of the same patient will provide invaluable information to advance individualised treatment strategies in cancer patients.
Cell-secreted microvesicles (exosomes) are the carrier of extracellular miRNAs
Extracellular miRNA, as a major RNA component of exosomes, was first discovered in 20078. Both mRNAs (poly-adenylated) and miRNAs, but not DNA, were identified in the exosomes of mast cells in this study, and were functionally transferable to other cells. These extracellular RNAs were therefore termed “exosomal shuttle RNA” (esRNA), and as such represent a novel mechanism of exchange of genetic information between cells. Exosomes are small (30-100 nm) membrane encapsidated vesicles of endocytic origin that are released into the extracellular environment by many cell types, including reticulocytes, immune cells, epithelial cells and tumour cells. Exosomal RNAs are heterogeneous in size but seem to be enriched in small RNAs. Their resistance to RNase and trypsin indicates an interior location in exosomes. Unlike intracellular RNA content, there are little or no ribosomal RNAs detected in exosomes. More than 120 miRNAs have been identified from exosomes of mast cells, including some involved in developmental timing (lin-4 and let-7) and some implicated in angiogenesis, hematopoiesis, exocytosis and tumuorigenesis (miR-1, miR-17, miR-18, miR-181 and miR-375). These exosomal miRNAs originate from a selective number of genes, and some are packaged exclusively into exosomes, suggesting a unique mechanism of RNA enrichment during the formation of exosomes. Given the extensive regulatory capacity of miRNAs and the board spectrum of target mRNAs each miRNA can affect upon transfer to another cell, discovery of exosomal miRNAs and their potential roles in intercellular communication are especially intriguing. A subsequent study confirmed the abundant presence of miRNAs in microvesicles of embryonic stem cells, which can be transferred to mouse embryonic fibroblasts9. Further, these microvesicles can be engineered to carry exogenous RNA and protein, such as GFP, and can transfer these contents to other embryonic stem cells, which suggests a novel therapeutic tool for delivering mRNA, miRNAs, protein, and siRNA to target cells.
Secretion of exosome encapsulated miRNAs from cancer cells has also been demonstrated. Using primary tumour cells from human glioblastoma tissues, Skog and Breakefield et al.10 indicated that cancer-released exosomes contain mRNA, miRNA and angiogenic proteins, and can be taken up by normal brain microvascular endothelial cells10. Thus, this cell-to-cell communication mechanism using exosomes as the transfer vehicles may represent a novel means of communication between cancer cells and the tumour microenvironment. It may also contribute to an autocrine stimulation of cancer growth, via the self-uptake of secreted exosomes. Microarray analysis was carried out to profile exosomal mRNAs, but not miRNAs in this study. More than 4000 different mRNAs were exclusively distributed in exosomes, suggesting a highly selective enrichment process during exosome formation. Ontologies of the glioblastoma exosomal mRNAs revealed pathways crucial in angiogenesis, cell proliferation, immune response, cell migration and histone modification, functions that may be involved in modifying the tumour stromal environment. Unlike mRNAs, several glioblastoma-specific miRNAs assessed by quantitative reverse transcription PCR seem to have parallel distributions in exosomes and in glioblastoma cells. Future thorough profiling of cancer-secreted miRNAs by microarray or deep sequencing analyses will increase our knowledge about the repertoire of exosome encapsulated miRNAs.
Circulating miRNAs are potential diagnostic biomarkers
Existence of cancer-derived exosomes in the peripheral circulation of cancer patients has been known for over 30 years11. Although some normal cells also secrete exosomes and may contribute to the level of exosomes in blood, the primary source of circulating exosomes in cancer patients is still cancer cells12,13. Immediately following the discovery of miRNA as a regular component of exosomes, cancer-derived circulating miRNAs were confirmed in the serum, plasma or whole blood samples from patients with prostate, ovarian and breast cancers by profiling or gene-specific PCR detection14-16. In one of these studies, cancer-derived exosomes were isolated by their affinity to the anti-epithelial cell adhesion molecule (EpCAM), as previous studies have demonstrated that exosomes from epithelial tumours express EpCAM on their surface. Interestingly, the levels of EpCAM-positive circulating exosomes are higher in patients with ovarian cancer compared to healthy controls, and increase along with the stage of cancer. Similar to the composition of exosomal RNAs, small RNAs represent the most abundant population of circulating RNAs, whereas the ribosomal RNAs are absent. Among the 218 miRNAs that have been detected in cancer and serum samples, 31 showed greater abundance in serum than in the corresponding tumours. Circulating miRNAs from ovarian cancer patients exhibit similar patterns that are distinct from benign ovarian disease and normal controls. Levels of 8 diagnostic miRNAs which are elevated in ovarian cancers compared to normal controls, including miR-21, -141, -200a, -200c, -200b, -203, -205 and -214, were all higher in the circulation of ovarian cancer patients compared to healthy donors15. Similarly, the serum level of miR-141, an miRNA specifically expressed in prostate cancer, can distinguish patients with prostate cancer from healthy controls14. In addition, cancer-derived circulating miRNAs may also reflect biopathologic features, such as miR-155, which is present at higher levels in the serum of patients with progesterone receptor (PR)-positive breast cancers compared to patients with PR-negative cancers16.
In a more recent study, a pan-human miRNA, high density microarray was successfully carried out using RNA samples isolated from one milliliter of serum of patients with prostate, colon, ovarian, breast and lung cancers. The results indicate that the overall serum miRNA levels are higher in cancer patients as compared to normal donors. Fifteen miRNAs (miR-16, -92a, -103, -107, -197, -34b, -328, -485-3p, -486-5p, -92b, -574-3p, -636, -640, -766, -885-5p) were elevated in sera from late-stage prostate cancer patients when compared to normal controls. Further, using a classifier of 28 miRNAs, heat map and cluster analyses successfully discriminated serum samples from normal versus cancer patients17. Future investigations on the correlations between cancer-derived circulating miRNAs and tumour traits, such as the response to therapy, will provide valuable diagnostic information and guide clinical decision making by enhancing our ability to predict drug response in cancer patients. Since some miRNAs may exclusively exist in circulating exosomes with low or undetectable levels in cancer cells, and since these may include unknown miRNAs, powerful discovery approaches such as deep sequencing analysis will be necessary to identify novel circulating miRNA markers.
Correct the malignant message from cancer – a hint in anticancer therapy
Cancer-secreted miRNAs can potentially carry out mRNA regulatory function in neighbouring cells and, most likely, transform the tumour microenvironment to assist tumour progression and affect tumour response to anti-cancer treatments. Upon entering the circulation, cancer-secreted miRNAs may further affect immune cells and cells at metastatic sites, once they attach and fuse to these cells. Therefore, a novel strategy to treat cancer is to therapeutically target these traveling miRNAs to cut off the “inside-out” signals initiated by cancer cells and their communications with surrounding cells that result in enhanced oxygen and nutrient supplies (via vascular endothelial cells), growth stimulation and invasion (via stromal fibroblasts) and immunosuppression (via immune cells). Targeting the crosstalk between cancer cells and their surrounding environment may also enhance the efficacy of existing chemotherapies and gene-targeted therapies through simultaneously affecting tumour cells and their microenvironment. Further elucidation of cancer-secreted miRNAs with functions in modifying the cancer niche and in drug resistance will precisely define the targets of these novel therapies. Possible therapeutic approaches include blocking the hyperactive exosomal secretion by cancer cells and delivering inhibitory therapeutic small RNAs, in order to inhibit the production and function of cancer-released miRNAs, respectively. These will also result in reduction or functional inhibition of cancer-derived miRNAs in the peripheral circulation of patients, which will block any distal effects of the circulating miRNAs while simultaneously allowing monitoring of the efficacy of treatment protocols. The systemic circulation of exosomes and, their non-immunogenic nature and ability to deliver nucleic acids and proteins in stable and functional forms, demonstrate a unique potential to engineer these particles as therapeutic vectors. Since exosomes interact with recipient cells through specific ligand-receptor interactions, this type of cell-to-cell transfer of genetic information should be cell type-specific, making it possible to design target-specific delivery of therapeutic molecules. In-depth mechanistic studies will be required to realise the prospect of engineering cells that are highly competent in secreting exosomes with therapeutic functions, which could serve as a novel approach for miRNA/siRNA and gene based therapies.
References
- Lee RC, Feinbaum RL, Ambros V (1993). The C. elegans heterochronic gene lin-4 encodes small RNAs with antisense complementarity to lin-14. Cell 75: 843-54.
- Calin GA, Croce CM (2006). MicroRNA signatures in human cancers. Nat Rev Cancer 6: 857-66.
- Iorio MV, Ferracin M, Liu CG, Veronese A, Spizzo R, Sabbioni S et al (2005). MicroRNA gene expression deregulation in human breast cancer. Cancer Res 65: 7065-70.
- Calin GA, Sevignani C, Dumitru CD, Hyslop T, Noch E, Yendamuri S et al (2004). Human microRNA genes are frequently located at fragile sites and genomic regions involved in cancers. Proc Natl Acad Sci U S A 101: 2999-3004.
- Kota J, Chivukula RR, O’Donnell KA, Wentzel EA, Montgomery CL, Hwang HW et al (2009). Therapeutic microRNA delivery suppresses tumorigenesis in a murine liver cancer model. Cell 137: 1005-17.
- Rossi JJ (2009). New hope for a microRNA therapy for liver cancer. Cell 137: 990-2.
- Shimono Y, Zabala M, Cho RW, Lobo N, Dalerba P, Qian D et al (2009). Downregulation of miRNA-200c links breast cancer stem cells with normal stem cells. Cell 138: 592-603.
- Valadi H, Ekstrom K, Bossios A, Sjostrand M, Lee JJ, Lotvall JO (2007). Exosome-mediated transfer of mRNAs and microRNAs is a novel mechanism of genetic exchange between cells. Nat Cell Biol 9: 654-9.
- Yuan A, Farber EL, Rapoport AL, Tejada D, Deniskin R, Akhmedov NB et al (2009). Transfer of microRNAs by embryonic stem cell microvesicles. PLoS One 4: e4722.
- Skog J, Wurdinger T, van Rijn S, Meijer DH, Gainche L, Sena-Esteves M et al (2008). Glioblastoma microvesicles transport RNA and proteins that promote tumour growth and provide diagnostic biomarkers. Nat Cell Biol 10: 1470-6.
- Taylor DD, Homesley HD, Doellgast GJ (1980). Binding of specific peroxidase-labeled antibody to placental-type phosphatase on tumor-derived membrane fragments. Cancer Res 40: 4064-9.
- Sabapatha A, Gercel-Taylor C, Taylor DD (2006). Specific isolation of placenta-derived exosomes from the circulation of pregnant women and their immunoregulatory consequences. Am J Reprod Immunol 56: 345-55.
- Taylor DD, Gercel-Taylor C (2005). Tumour-derived exosomes and their role in cancer-associated T-cell signalling defects. Br J Cancer 92: 305-11.
- Mitchell PS, Parkin RK, Kroh EM, Fritz BR, Wyman SK, Pogosova-Agadjanyan EL et al (2008). Circulating microRNAs as stable blood-based markers for cancer detection. Proc Natl Acad Sci U S A 105: 10513-8.
- Taylor DD, Gercel-Taylor C (2008). MicroRNA signatures of tumor-derived exosomes as diagnostic biomarkers of ovarian cancer. Gynecol Oncol 110: 13-21.
- Zhu W, Qin W, Atasoy U, Sauter ER (2009). Circulating microRNAs in breast cancer and healthy subjects. BMC Res Notes 2: 89.
- Lodes MJ, Caraballo M, Suciu D, Munro S, Kumar A, Anderson B (2009). Detection of cancer with serum miRNAs on an oligonucleotide microarray. PLoS One 4: e6229.
About the authors
Dr John Rossi
Dr Rossi is the Lidow family Professor and Chair of the Division of Molecular Biology, Beckman Research Institute of the City of Hope, and Dean, Irell and Manella Graduate School of Biological Sciences, Beckman Research Institute of the City of Hope. Dr Rossi received his doctoral training in genetics at the University of Connecticut in Storrs and postdoctoral training in molecular genetics at Brown University. His research has focused on RNA biology and clinical applications of small RNAs. His group was the first to demonstrate that hammerhead ribozymes could be used for inhibition of HIV replication. This research program led to two clinical trials in which ribozyme genes have been transduced into hematopoietic stem cells for autologous transplant in HIV infected individuals. He is the recipient of an NIH Merit award for his work on ribozymes and HIV. Work in the laboratory continues to focus upon mechanisms of small RNA mediated inhibition of gene expression and RNA based therapeutics, with recent emphasis on function and applications of RNA interference and expressed short hairpin RNAs for therapeutic treatment of HIV and cancers. He has published over 200 peer reviewed articles and numerous reviews and commentaries on RNAi based therapeutics.
Emily Wang
Dr Wang, Assistant Professor in the Division of Tumour Cell Biology, Beckman Research Institute of the City of Hope, first began her research career in the late 1990s as a virologist. She obtained her doctorate from University of Nebraska-Lincoln and Nankai University in China in 2002, followed by a post-doctoral training in viral oncology in the laboratory of Dr Gary S Hayward at the Sidney Kimmel Cancer Center of Johns Hopkins School of Medicine. In late 2003, Dr Wang started another post-doc tenure in the laboratory of Dr Carlos L Arteaga at the Vanderbilt-Ingram Cancer Center, where she became a committed cancer researcher with a breast cancer focus. During her fellowship at Vanderbilt, Dr Wang received a K99/R00 Pathway to Independence Award from NIH/NCI for her studies on the crosstalk between TGF-beta and HER2 in breast cancer. She was also awarded the American Association for Cancer Research (AACR) Scholar-in-Training Award. In 2008, Dr Wang started a tenure-track Assistant Professor position at City of Hope, where she and her laboratory are investigating cellular and molecular mechanisms of oncogenic signaling in breast carcinogenesis. Dr Wang has approximately 40 publications related to her research in cancer and viral oncology.