Evolution and revolution in time-of-flight mass spectrometry and its impact on research within the pharmaceutical industry
Posted: 20 June 2011 | Tony Bristow (AstraZeneca)
Time of flight mass spectrometry (TOF-MS) has been an attractive choice of instrument for many years due to its potentially unlimited m/z range, high-speed acquisition, accurate mass measurement capability and sensitivity. Originally commercialised in the late 1950’s by the Bendix Corporation1, several physical and technical issues of the early TOF instruments limited both mass resolving power and mass accuracy2. From the early 1970’s to the early 1990’s, these limitations were overcome. Initially the advent of reflectron TOF-MS overcame the ion energy spread, hence increasing mass resolution3. The later combination with orthogonal acceleration (oa) can be seen as the catalyst for the vast range of TOF instrumentation that is available today, with greatly improved mass resolving power and mass accuracy4,5.
Commercial development of oa-TOF-MS and QTOF-MS was led by Micromass (later Waters) and Applied Biosystems (AB, now AB SCIEX) with instruments such as the QTOF-1, the LCT, the Mariner and the QSTAR. With instrumentation of this type, mass resolving power as high as 5000 (FWHM) and typical mass accuracy of < 10 ppm was possible6. These instruments were initially designed for hyphenation with liquid chromatography, however the natural development of an accurate mass gas chromatography-oa-TOF-MS instrument soon followed with the launch of the GCT by Micromass and later the Jeol Accutof. Though outside the main scope of this article, the author also refers readers to the development of the delayed extraction (DE) technique for increased resolution and improved mass accuracy with matrix-assisted laser desorption/ionisation (MALDI) TOF-MS7.
For a number of years following the original commercial launch in 1996/1997, both oa-TOF-MS and QTOF-MS technology were only available from Micromass and AB. However, in the last decade we have witnessed the emergence of other vendors in both the oa-TOF and QTOF-MS markets. This has not only increased commercial competition, but has also driven innovation in the technology and has resulted in a great leap forward in capability, in fact a revolution in TOF-MS. Instruments are now also available from Agilent Technologies, Jeol, Bruker Daltonics, Shimadzu, Perkin Elmer and LECO. The timeline of this evolution can be described and illustrated in terms of increasing in mass resolving power coupled with the improved mass accuracy. Figure 1A shows the increasing mass resolving power of the commercially available instruments from 1996 to the present. The plot is also extrapolated (from 2011 to the arbitrary year of 2015) to the mass resolving power of the prototype instruments developed in academia8. Figure 1B (page 14) shows the improvement in mass accuracy over the same time period. It is of interest to compare this data to that from an independent inter-laboratory study on accurate mass measurement published in 20039. This study showed typical mass accuracy of the oa-TOF-MS and QTOF-MS instruments of 5 ppm and below. Within the timeline described in Figure 1, a number of key innovations are of note.
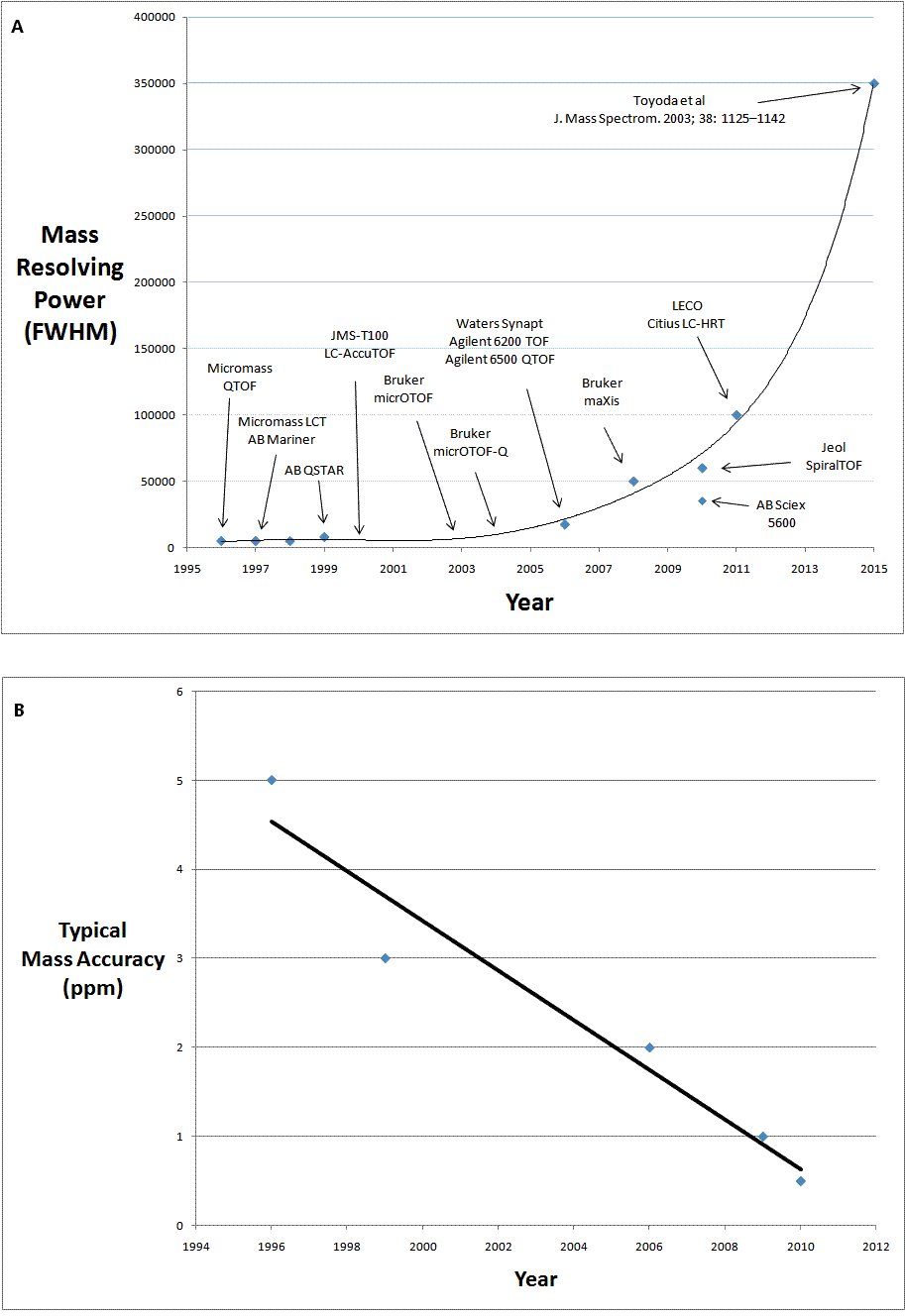
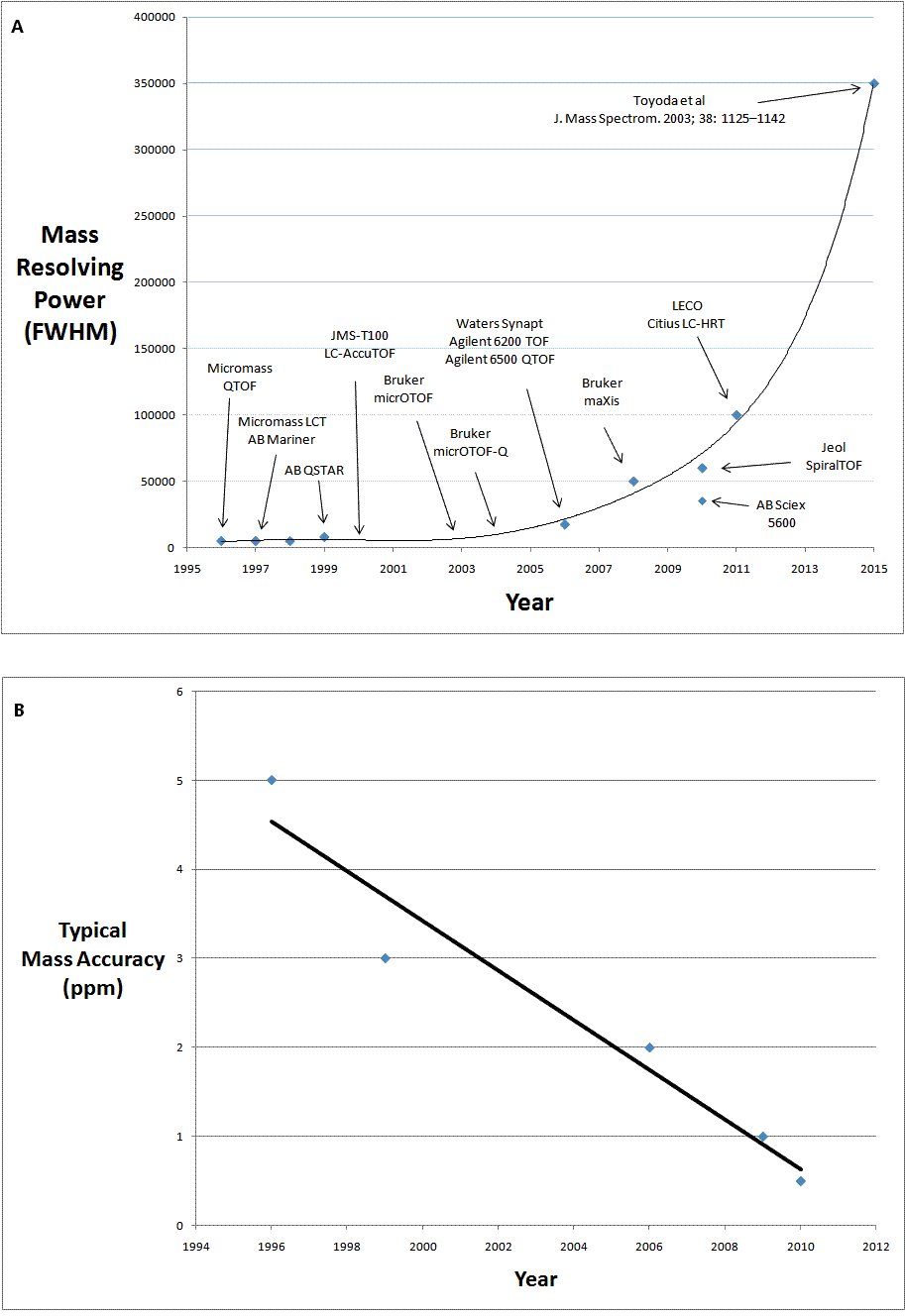
Figure 1 (A) the increase in mass resolving power of commercially available instruments from 1996 to the present and (B) the improvement in mass accuracy over the same time period
Bruker maXis
Figure 1 shows a fairly linear increase in the mass resolving power of commercially available oa-TOF and QTOF-MS instrumentation up to 2006 as more vendors came to the market. The launch of the Bruker maXis in 2008 provided a step change in performance with a mass resolving power in excess of 40000 (FWHM). In 2011, Bruker’s next generation instrument, the maXis 4G, was launched with an even greater resolving power of 60000 (FWHM).
Waters Synapt
The Synapt was a revolutionary combination of QTOF-MS technology and ion mobility based separation. This combination affords gasphase analyte characterisation based on both m/z ratio and gas-phase ion mobility separation (drift time). Launched originally in 2006 (mass resolving power of 17500 (FWHM) and mass accuracy of 3 ppm), the specifications were enhanced in 2009 with the next generation Synapt G2 with mass resolving power as high as 40000 (FWHM) and mass accuracy of 1 ppm.
Jeol JMS-S3000 Spiral TOF
Multiturn TOF-MS technology has been shown to have mass resolving power in excess of 3500008. This is a result of mass resolution being directly proportional to total flight path length. In 2010, this academic promise became commercial reality with the launch of the JMS-S3000 SpiralTOF, with a mass resolving power specification in excess of 60000.
Shimadzu LCMS-IT-TOF
Shimadzu produced a unique hybrid mass spectrometer with the combination of an ion trap and TOF-MS and this came to the market in 2004. The instrument is capable of multiple stages of tandem mass spectrometry experiments (MSn) in combination with accurate mass measurement.
AB SCIEX TripleTOF 5600
In 2010, AB SCIEX re-entered the QTOF-MS market with the TripleTOF 5600. Like all QTOFs it is an instrument capable of structural characterisation and the 5600 has much improved mass resolving power over earlier AB SCIEX instruments (mass resolving power of 35000, mass accuracy of 1 ppm). Uniquely from launch, AB SCIEX have also emphasised the quantitative capability of this new instrument, which is an area where both oa-TOF-MS and QTOF-MS technology have not been broadly applied to date.
LECO Citius LC-HRT and the Pegasus GC-HRT
In 2011, the capability of oa-TOF-MS was enhanced even further with the launch of two instruments by LECO. By employing a multiple pass technology (Folded Flight Path™), mass resolving powers of 100,000 FWHM have been demonstrated for the Citius LC-HRT and 50,000 FWHM for the Pegasus GC-HRT. Again, it is a multiple pass approach that provides a greatly enhanced total flight path length and hence this much increased mass resolving power. Both instruments have been shown to have mass accuracy of
Both oa-TOF-MS and QTOF-MS technology were adopted within the pharmaceutical industry from its initial launch as a tool for structural characterisation in all areas of drug discovery and development and continues to be applied extremely widely. A thorough literature review is beyond the scope of this article, therefore a number of publications have been selected to illustrate the adoption and impact of the developments in TOF technology.
One of the first publications demonstrating the potential for the application of LC-QTOF came from Eckers et al in 199710. The researchers described the application of accurate mass LC-MS/MS for the structural characterisation of trace impurities in cimetidine, with typical mass accuracy of < 10 ppm. In 2001, Wolff et al presented data resulting from an improved experimental set-up on a QTOF which employed a dual spray ion source11. The development enabled the authors to demonstrate improved mass accuracy in the range 1.5-2.5 mDa in both MS and MS/MS experiments. In 2001, Neville Haskins outlined the state-of-the-art in oa-TOF-MS at that time12. In the article, the impact of oa-TOF-MS technology on acquisition speed, sensitivity, mass resolving power and mass accuracy were emphasised along with the benefits to pharmaceutical research.
As described earlier, one of the key technical developments in QTOF technology over the past 15 years was its combination with ion mobility, resulting in the commercial launch of the Synapt series of instruments. The potential impact of this technology on the pharmaceutical industry was demonstrated by Eckers and co-workers13. Their 2007 publication described the detection of trace impurities in formulated drug products. In the study, the separation from polyethylene glycol (PEG) based excipients from drug related impurities by IMS and detection by TOF-MS was exemplified.
Oa-TOF-MS and QTOF-MS technology has also been widely applied in the area of drug metabolism and pharmokinetics. Eva Lens and colleagues at AstraZeneca demonstrated the application of UPLC/oa-TOF-MS (and independently 1H NMR) in a metabonomic study14. Examination of the data aided by statistical interpretation made it possible to identify potential markers for toxicity of pravastatin. Temesi and colleagues have also demonstrated the application of oa-TOF-MS in metabolic studies15. The main focus of their work was to understand the potential of the technology in a quantitative application traditionally dominated by triple quadrupole mass spectrometry. For specificity narrow m/z window, extracted ion chromatograms were employed and the identities of target metabolites were confirmed.
Oa-TOF and QTOF-MS continue to find new pharmaceutical applications. An interesting example is the work of Harry et al, which demonstrated the potential of ion mobility in combination with MS for real-time reaction monitoring16. The use of IMS-MS analysis was demonstrated for the monitoring of the reaction products formed when 7-fluoro-6-hydroxy-2- methylindole is deprotonated by aqueous sodium hydroxide, with the reaction mixture sampled and analysed at intervals of several minutes. The combined IMS-MS approach showed potential as a rapid and selective technique to aid pharmaceutical process understanding.
Future perspectives
Theoretically, TOF instrumentation has even more potential, with mass resolving power of 350000 already demonstrated on prototype instruments based on multi-turn ion optical geometries8. This increase in resolving power coupled with improved mass accuracy will allow oa-TOF-MS and QTOF to provide data of ever increasing quality for structural characterisation. This leads to even greater confidence and certainty in the conclusions made from such data. The quantitative application of both oa-TOF-MS and QTOF instrumentation will continue to be developed. Finally, the capability of both oa-TOF-MS and QTOF for high speed data acquisition makes both techniques ideal partners for UHPLC, with ever shorter run times and narrower peak widths.
Acknowledgements
I would like to thank the following people for providing technical information for this article. Brian Williamson and Lydia Nuwaysir (AB SIEX), Jason Wildgoose (Waters), Tim Smith (Jeol), George McCleod (Bruker Daltonics), Ashley Sage (Agilent Technologies), Jeffrey Patrick (LECO) and Alessandro Baldi and Nicola Vosloo (PerkinElmer). I would also like to thank John Langley (University of Southampton) for providing advice and comments on the article.
References
- Wiley WC, McClaren IH. 1955. Time-of-flight mass spectrometer with improved resolution. Rev Sci Instrum. 26:1150–1157
- Cotter RJ. 1992. Time-of-flight mass spectrometry for the structural analysis of biological molecules. Anal Chem. 64:1027A–1039A
- Mamyrin BA, Karataev VI, Shmikk DV, Zagulin VA. 1973. Mass reflectron. New nonmagnetic time-of-flight high-resolution mass spectrometer. Zhurnal Eksperimental’noi i Teoreticheskoi Fiziki. 64(1): 82-89
- Dawson JHJ, Guilhaus M. 1989. Orthogonalacceleration time-of-flight mass spectrometer. Rapid Commun in Mass Spectrom. 3(5): 155-159
- Coles J, Guilhaus M. 1993. Orthogonal acceleration – a new direction for time-of-flight mass spectrometry: fast, sensitive mass analysis for continuous ion sources. Trends in Analytical Chemistry. 12(5): 203-213
- Morris HR, Paxton T, Dell A, Langhorne J, Berg M, Bordoli RS, Hoyes J, Bateman RH. 1996. High Sensitivity Collisionally-activated Decomposition Tandem Mass Spectrometry on a Novel Quadrupole/Orthogonalacceleration Time-of-Flight Mass Spectrometer. Rapid Commun in Mass Spectrom. 10: 889-896
- Vestal ML, Juhasz P, Martin SA. 1995. Delayed Extraction Matrix-assisted Laser Desorption Time-of- Flight Mass Spectrometry. Rapid Commun Mass Spectrom. 9: 1044-1050
- Toyoda M, Okumura D, Ishihara M, Katakuse I. 2003. Multi-turn time-of-flight mass spectrometers with electrostatic sectors. J. Mass Spectrom. 38: 1125–1142
- Bristow AWT, Webb KS. 2003. Intercomparison study on accurate mass measurement of small molecules in mass spectrometry. J. Am. Soc. Mass Spectrom. 14: 1086-1098
- Eckers C, Haskins N. 1997.The use of liquid chromatography combined with a quadrupole timeof- flight analyser for the identification of trace impurities in drug substance. Rapid Commun in Mass Spectrom. 17: 1916–1922
- Wolff JC, Eckers C, Sage AB, Giles K, Bateman R. 2001. Accurate Mass Liquid Chromatography/Mass Spectrometry on Quadrupole Orthogonal Acceleration Time-of-Flight Mass Analyzers Using Switching between Separate Sample and Reference Sprays. 2. Applications using the Dual-Electrospray Ion Source. Anal Chem. 73: 2605-2612
- Haskins NJ. 2001. Faster compound analysis by mass spectrometry – the ToF revolution. Journal of Pharmaceutical and Biomedical Analysis. 25: 767-773
- Eckers C, Laures AMF, Giles K, Major H, Pringle S. 2007. Evaluating the utility of ion mobility separation in combination with high-pressure liquid chromato – graphy/mass spectrometry to facilitate detection of trace impurities in formulated drug products. Rapid Commun in Mass Spectrom. 21: 1255–1263
- Lenza EM, Williams RE, Sidaway J, Smith BW, Plumb RS, Johnson KA, Rainville P, Shockcor J, Stumpf CL, Granger JH, Wilson ID. 2007. The application of microbore UPLC/oa-TOF-MS and 1H NMR spectroscopy to the metabonomic analysis of rat urine following the intravenous administration of pravastatin. Journal of Pharmaceutical and Biomedical Analysis. 44 :845-852
- Temesi DG, Martin S, Smith R, Jones C, Middleton B. 2010. High-throughput metabolic stability studies in drug discovery by orthogonal acceleration time-offlight (OATOF) with analogue-to-digital signal capture (ADC). Rapid Commun in Mass Spectrom. 24: 1730–1736
- Harry EL, Bristow AWT, Wilson ID, Creaser CS. 2011. Real-time reaction monitoring using ion mobilitymass spectrometry. Analyst.136: 1728-1732
About the Author
Tony Bristow gained his degree in Applied Chemistry from Sheffield Polytechnic in 1992. He then carried out a PhD on MALDI- ion trap mass spectrometry under the supervision of Professor Colin Creaser at Nottingham Trent University. Tony moved to Kodak Limited in Harrow in 1995, working in both mass spectrometry and separation science. Following a secondment from Kodak at LGC in Teddington, he joined LGC in 2002. At LGC, Tony worked on a variety of mass spectrometry based projects, mainly focused on FT-ICRMS, accurate mass measurement and MS/MS.
In November 2005, Tony joined AstraZeneca where he works in Pharmaceutical Development at Macclesfield, focusing on the application of mass spectrometry at all stages in drug development. He has published many peer-reviewed articles on his research in mass spectrometry and a number of other analytical techniques. He is a regular presenter at both national and international mass spectrometry conferences and an active member of the British Mass Spectrometry Society (BMSS) committee and currently holds the post of Vice-Chair.
Email: [email protected]