Spray drying pharmaceuticals
Posted: 13 December 2011 | | No comments yet
Spray drying is a widely used technical method to produce fine particles, coarse powders, agglomerates or granulates in various industries. The characteristics of the particles produced by this method can be controlled and the particle properties can be maintained as constant throughout a continuous operation. The product from this process can readily meet the product specifications that are most desirable for subsequent processing or direct application. Hence, it has been successfully applied in the pharmaceutical industry to process both primary pharmaceuticals like APIs and also the final pharmaceutical formulations. The aim of this review is to present current pharmaceutical applications of this method and share my perspectives from both an industrial and academic point of view. I hope that it can serve as inspiration for some peers in pharmaceutical field.
By definition, spray drying is the transformation of a feed from a fluid state into a dried particulate form by spraying the feed into a hot drying medium1. The drying medium is typically air, but an inert gas, e.g. nitrogen, can be employed when the liquid is a flammable solvent or the product is oxygen-sensitive. It is a one-step, continuous particle formation process involving drying. The feed can be a solution, suspension, emulsion, dispersion or even paste, and the solvent medium can either be aqueous or organic. The dried product from the process conforms to particles, powders, agglomerates or granules, and the form of which depends upon the physical and chemical properties of the feed, the dryer design and the operation conditions1.
There are three major phases involved in the spray drying process. Firstly, the feed is atomised into fine spray in a drying chamber. Then the fine spray of the feed comes into contact with a hot drying gas and the evaporation of liquid takes place spontaneously, resulting in dry particles. Finally, the dried particles are separated from the gas by using a cyclone and / or a filter. When the feed and the hot drying gas are mixed upon atomisation, the flow of the feed and the gas can be co-current, mixed-flow or counter-current (Figure 1). Co-current drying is the most commonly used technique due to its flexibility and low risk of heat damaging the product. Mixed-flow dryers are typically equipped with either a fountain nozzle system or a combined spray dryer – fluid bed dryer system. The former allows larger particles to be produced in a more compact plant and the product is heavy enough and thermally stable. The added complexity of the combined spray dryer – fluid bed dryer system allows more particle engineering options through agglomeration without the requirement of thermal stability of final products. The countercurrent mode is very rarely used in the pharmaceutical industry and would only be used for coarse materials when the substances are thermally stable, and when a high thermal load is needed to dry the substances. The feed must be dispersed into a spray with a controlled droplet size distribution using an appropriate atomiser. The most commonly used atomiser / spray nozzles are the rotary disk atomiser and pressurised nozzle, which is suitable for large scale manufacturing of dry products with a large particle size. For a small scale manufactur – ing or when finer particles are required, usually a two-fluid nozzle or sometimes an ultrasonic nozzle is used. The atomisation condition and con figuration of spray nozzle are usually considered to be the dominant parameter controlling final particle size distribution since the size of the spray-dried particles is mainly dependent on the size of the initial droplet size in non-agglomerating spray dryers.
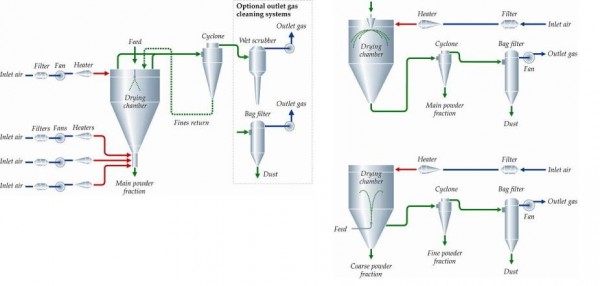
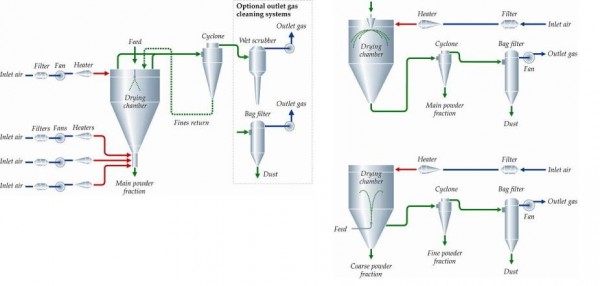
FIGURE 1 Illustration of different flow of the feed and the gas in spray dryer. A, Co-current; B, mixed flow – combined spray dryer – fluid bed dryer system; C, mixed flow – fountain nozzle system Copyright: GEA Niro
In the spray drying process, the drying rate of the feed is extremely fast, because the feed can be atomised into a fine spray with a huge surface area that facilitates evaporation of the solvent. The drying of the droplets in this process can be accomplished within a time range of milliseconds to a few seconds. The evaporation leads to a cooling of the droplets and thus just a very small thermal load put on to dry particles. The dry particles leave the drying chamber following the flow of drying gas, so the resi – dence time of the dried particles in the chamber depends on the flow rate of the drying gas, the flowability of the powder and dimension of the drying chamber. Usually when the desired removal of moisture is completed, the dry product is removed from the dryer before the product temperatures rise to the outlet temperature. Hence, there is little likelihood of heat damage to the product2.
In spite of the short drying time, spray drying allows the formation of dry particles, and the qualities of the particles are determined by the interplay between the evaporation rate of the solvent and the diffusion rate of solute in the droplets during the evaporation3. The particle formation mechanisms during the spray drying process have been intensively studied in past decades and tremendous know-how knowledge has been obtained4-6. Nevertheless, spray drying still presents a wide field for research and development for interested pharmaceutical scientists and technologists, and continuously amazes with the huge potential of particle engineering by adjusting the factors in the spray drying process such as the humidity of drying gas, inlet and outlet temperature, atomising gas flow rate (atomisation energy), feed rate, solid concentration in the feed, viscosity of the feed, physicochemical properties of the solid in the feed and co-solvent system etc7. As importantly, the process can be scaled basically to any drying gas flow rate with the right equipment.
Pharmaceutical application of spray drying: application in APIs or excipients
Spray drying process can render a dried product with desirable characteristics for subsequent processing e.g. direct compression. As an example, spray-dried lactose was introduced to the pharmaceutical market in the 1960s as an excipient that enables direct compression of formulations in a simple manufacturing process8. Prior to the spray drying process, lactose can be suspended in a saturated aqueous solution. The drying process converts them into free flowing granules with a mixed solid state of crystal form and amorphous form. The resulting unique internal structure can confer the dry powder a good plasticity and binding during the process of direct tableting. To date, lactose remains one of the most popular excipients for active pharmaceutical ingredients (APIs) whose dose makes them suitable for direct compression. Other direct compressible excipients produced from spray drying include EMDEX (spray dried dextrose), Avicel HFE-102 (co-spray dried microcrystalline cellulose and mannitol), Karion Instant (spray dried sorbitol), TRI-CAFOS (spray-dried tricalcium phosphate) and Advantose 100 (spray-dried maltose)9. Besides excipients, APIs can also be spray-dried in order to obtain some of following properties such as improved flowability, adherence or agglomeration for tableting and wettability in water. Due to the almost instantaneous transition between liquid and solid phases, spray drying usually results in predominately amorphous material for small molecule substances, especially when particle temperature during the process is typically lower than the glass transition temperature of the material. This is often desirable, as it may be used to increase the bioavailability of the resulting drug product. However, the physicochemical stability of the dry product is always a concern due to the high tendency of transformation from metastable amorphous form to stable solid form. Hence, a suitable packaging has to be applied to minimise the physicochemical degradation of the drug product during its shelf life.
Application in pharmaceutical formulations: solid dispersion
APIs are often co-spray dried with some excipients in order to achieve desirable functionality for the final pharmaceutical formulations. Producing solid dispersion by spray drying may be one of the main applications of this technique in the pharmaceutical industry. Nowadays, approximately 40 per cent of NCEs have low solubility in water, and possess poor bioavailability. One of the strategies to improve oral absorption is to improve the dissolution rate of the NECs by formulating them into solid dispersion. Spray drying and melt-quenching are regarded as the two most established approaches that can highly disperse the poorly-water soluble drug substances into solid dispersion carriers (mostly polymeric matrix). Nevertheless, as compared to melt-quenching, the thermal decomposition of drugs or carriers can be prevented by using spray drying since solvent is evaporated rapidly and dry product undergoes a low temperature10.
A general procedure of preparing solid dispersion using spray drying consists of dissolving the drug and polymeric carrier in an organic solvent, e.g. ethanol, chloroform, or a mixture of ethanol and dichloromethane11, then spraying it into a stream of heated nitrogen gas flow to remove the organic solvent. The use of organic solvents, the high preparation cost and the difficulties in completely removing the solvent are some of the disadvantages associated with this method10. Moreover, physical stability of molecularly dispersed drugs still presents a major challenge in the development of solid dispersions12.
Microencapsulation
The goals of microencapsulation in the pharmaceutical industry are to stabilise active compounds, mask the bitter taste of the drug substance or design modified release pharmaceutical formulations. Through selecting suitable excipients and feed solvents, the active drug substances can be encapsulated by a polymer shell or coat13. The feed can be an emulsion, suspension or polymer solution containing active substances. Microencapsulation can also be achieved through spray congealing where the materials are melted and sprayed into cold air to congeal the particles instead of being dissolved in a solvent medium prior to atomisation.
Taste masking
For bitter drug substances, a taste masking formulation will certainly improve patient compliance. Taste masking may be more significant when formulating an orally disintegrating tablet system for drug substances of this type. Bora et al developed taste-masked microspheres for the intensely bitter drug ondansetron hydrochloride by spray drying14. Both chitosan and Eudragit E100 were shown to mask the bitter taste of the drug but did not compromise the drug release. Sollohob et al also used spray drying to obtain the roxithromycin containing microcapsules with high taste masking efficiency, and Eudragit L30D-55 was chosen as a barrier coating15.
Modified release formulation
Modified release formulations designed using spray drying can be in a form of micro capsule, microsphere or microparticle. A microcapsule can be defined as an active substance which is covered by a shell or coat by forming a core-shell reservoir structure. The release of the drug substance from the microcapsule is mainly controlled by the diffusion rate of dissolved drug through the shell or the coat. In contrast, the drug release rate of microspheres or microparticles can be controlled by the dissolution rate of the drug or the carriers and diffusion rate of drug from an insoluble matrix. Microcapsules are more likely achieved by spray drying of an emulsion or a suspension rather than a solution. The excipients, often polymeric materials, dissolved in the dispersion phase may form a shell during spray drying to encapsulate the active substances. Microcapsule can render delayed release profiles to the drug, whereas microparticle and microsphere are more likely suitable for the design of sustained release formulations. The selection of one of these forms is dependent on the therapeutic aspects and physicochemical properties of the drug substance. Varshosaz et al developed colontargeted microcapsule of budesonide for ulcerative colitis by spray drying of the drug with dextran, and demonstrated that the microcapsules could target the drug to colon. And its efficacy in reducing macroscopic damage score was higher than mesalasine suspension16.
Dry powder for inhalation
An obvious advantage of spray drying over milling to produce dry powder for inhalation lies in its unique feature of particle engineering. Distinct from other formulations, for dry aerosol formulation, the particle characteristics are extremely important, which determine the lung deposition efficiency of the dry aerosols, thus their therapeutic efficacies. Even though technologies like supercritical fluid technology, spray freeze drying, crystal engineering, electrospray etc are emerging for particle design with different functionalities, spray drying is still regarded as the most adopted method in the pharmaceutical industry to produce dry aerosol formulations besides the milling process. Nevertheless, in terms of pulmonary delivery of biopharmaceuticals, spray drying may be more attractive than milling. In fact, the first inhalable insulin product, Exubera® for Type 1 and 2 Diabetes was produced by spray drying17.
When spray drying of biopharmaceuticals, cryo- or lyo-protectants such as disaccharides is often employed to not only protect the liable compounds from degradation during the process but also to render them desired particle properties. Most of the research has been focused on the effect of the disaccharides on the stability of the biopharmaceuticals. Less work has been done on investigating the effect of biopharmaceuticals on solid state of disaccharides, which influences physical stability of dry particles. Our recent research has clearly demonstrated that the polymorphic form of spray drying mannitol can be changed upon proteins and different drying rate. Further particle dependence of polymorphism in spray dried mannitol was also observed when a model protein lysozyme was added in the formulation18.
Aseptic production with spray drying
Aseptic spray drying production is required for producing dry aerosol formulations, injectable depot formulations and also biopharmaceutical formulations. Biopharmaceuticals, like protein and peptide drugs, have become more and more important classes of therapeutic agents in the pharmaceutical industry19. They are often kept in a solid form due to stability concern. Currently, lyophilisation is the most commonly used method to produce dry protein products. But it is time-consuming and a high energy consuming technique. In contrast, aseptic spray drying production, which is more economical in terms of installation and operation compared with lyophilisation, may be an alternative to producing dry protein product20. After all, spray drying is also superior to lyophilisation with respect to engineering particle property and facilitating subsequent processing. It should be mentioned that the only process difference of aseptic spray drying from non-aseptic spray drying is the requirements for sterilisation. However, different equipments are required20.
Other applications of spray drying in pharmaceutical research
Besides the aforementioned pharmaceutical applications, spray drying has also been attempted to design many other pharmaceutical formulations. For example, liposomal formulations21, lipid based self-emulsifying drug delivery systems22, dispersions of porous and non-porous silica23,24, solid-in-oil dispersion25, pre-prepared nanoparticle suspension and dry extracts of active raw materials from plants have also been treated using spray drying for various purposes like inhalation, controlled release, stabilisation, targeting drug delivery. In short, pharmaceutical scientists are continuously exploring to expand the pharmaceutical application of spray drying.
Perspectives of spray drying pharmaceuticals
Spray drying of pharmaceuticals is no longer just an approach to obtain dry product. The last decade has been a shift from empirical formulation efforts to a particle design approach based on a better understanding of particle formation in the spray drying process3,26. In order to design sophisticated drug delivery systems, particle engineering via spray drying has been one of the most studied areas in pharmaceutical research field. To this end, various experimental techniques like levitation techniques and droplet chain techniques have been introduced to investigate single droplet drying kinetics and the physical and chemical mechanisms that control particle formation, which is the key to successful particle engineering via this method. Additional innovation may lie in understanding biological processes of the newly engineered particles, e.g. in vivo release, cellular targeting, intracellular trafficking, drug absorption and biological compatibility.
From an industrial point of view, spray drying will slowly but surely become part of the pharmaceutical industry’s standard27. Further advances in spray drying may be achieved by combining the spray drying process with additional processing steps and the application of computational fluid dynamics (CFD)28. One of the obstacles in the pharmaceutical application of spray drying lies in the limited knowledge of this technology in many pharmaceutical companies. Luckily, more and more pharmaceutical organisations now realise both process and formulation equally determine critical quality attributes of drug products. Pharmaceutical technologists are starting to get involved in decision-making at an early stage of drug development, which may be able to bring this powerful technology into a full play in a short future.
Acknowledgement
The author would like to thank Henrik Schwartzbach of GEA Niro Chemical Division for his invaluable help with formulating the article.
About the Author
Dr. Mingshi Yang is an associate professor in the Department of Pharmaceutics and Analytical Chemistry of the University of Copenhagen. Before taking up this position in 2009, he had worked as a research scientist in Novo Nordisk A/S since 2006. He obtained his postdoctoral training in the Danish University of Pharmaceutical Sciences, Denmark and Gifu Pharmaceutical University, Japan, respectively. His research has been focused on solid formulations, with an emphasis on design of particulate delivery systems for both biomacromolecules and small molecules. One of his current research interests is on particle engineering via spray drying for biopharmaceuticals. His production as a researcher includes over 40 publications in scientific journals, reviews and international conferences, two invited lectures and a book chapter.
References
1. K. Masters, Spray drying handbook, 1991, by Longman Scientific & Technical. Wiley
2. Training Papers Spray Drying, Buchi LabortechnikAG, Switzerland; http://www.buchi.com/Spray-Drying. 69.0.html?&no_cache¼1&file¼308&uid¼2283
3. R. Vehring, Pharmaceutical Particle Engineering via Spray Drying. Pharmaceutical Research, 25 (2008) 999-1022
4. J. Broadhead, S.K. Edmond Rouan, and C.T. Rhodes, The spray drying of pharmaceuticals. Drug Development and Industrial Pharmacy, 18 (1992) 1169–1206
5. M.I. Ré. Formulating drug delivery systems by spray drying. Dry. Technol. 24 (2006) 433–446
6. R. Vehring, W. R. Foss, D. Lechuga-Ballesteros, Particle formation in spray drying, J. Aerosol Sci. 38 (2007) 728–746
7. K. Cal, K. Sollohub, Spray Drying Technique. I: Hardware and Process Parameters. J Pharm Sci. 99 (2010) 575-586
8. W. Gunsel and L. Lachman, Comparative Evaluation of Tablet Formulations Prepared from Conventionally Processed and spray-Dried Lactose. J. Pharm. Sci. 52 (1963) 178–182
9. M. C. Gohel, A review of co-processed directly compressible excipients. J Pharm Pharmaceut Sci. 8 (2005) 76-93
10. D.H. Won, M.S. Kim, S. Lee, J.S. Park, S.J. Hwang, Improved physicochemical characteristics of felodipine solid dispersion particles by supercritical anti-solvent precipitation process. Int. J. Pharm. 301 (2005) 199-208
11. P.A. Rivera, M.C. Martinez-Oharriz, M. Rubio, J.M. Irache, S. Espuelas, Fluconazole encapsulation in PLGA microspheres by spray-drying. J. Microencapsulation. 21 (2004) 203–211
12. J.X. Wu, M. Yang, F. van den Berg, J. Pajander, T. Rades, J. Rantanen, Influence of evaporation rate and formulation factors on solid dispersion physical stability. European J Pharm Sci. (2011)
13. K. Bowey, R.J. Neufeld, Systemic and mucosal delivery of drugs within polymeric microparticles produced by spray drying. Biodrug. 24 (2010) 359-377
14. D. Bora, P. Borude, K. Bhise, Taste Masking by Spray-Drying Technique, AAPS PharmSciTech, 9 (2008) 1159-1164
15. K. Sollohub, M. Janczyk, A. Kutyla, H. Wosicka, P. Ciosek, K. Cal, Taste masking of roxithromycin by spray drying technique, Acta Pol Pharm, 68 (2011) 601-604
16. J. Varshosaz, F. Ahmadi, J. Emami, N. Tavakoli, M. Minaiyan, P. Mahzouni, F. Dorkoosh, Micro – encapsulation of budesonide with dextran by spray drying technique for colon-targeted delivery: an in vitro/in vivo evaluation in induced colitis in rat. J Microencapsul. 28 (2011) 62-73
17. S. White, D.B. Bennett, S. Cheu, P.W. Conley, D.B. Guzek, S. Gray, J. Howard, R. Malcolmson, J.M. Parker, P. Roberts, N. Sadrzadeh, J.D. Schumacher, S. Seshadri, G.W. Sluggett, C.L. Stevenson, N.J. Harper, EXUBERA®: pharmaceutical development of a novel product for pulmonary delivery of insulin, Diabetes Technol. Ther.7 (2005) 896–906
18. Y.Y. Lee, J.X. Wu, M. Yang, P.M. Young, F. van den Berg, J. Rantanen, Particle size dependence of polymorphism in spray-dried mannitol. Eur. J. Pharm. Sci. doi:10.1016/j.ejps.2011.06.002. (2011)
19. M. Yang, S, Frokjaer, Delivery Technologies for Biopharmaceuticals: Peptides, Proteins, Nucleic Acids and Vaccines, John Wilely & Sons, Ltd, (2009)
20. H. Schwartzbach, Achieving aseptic drying with spray drying technologies. Pharmaceutical Technology Europe. Volume 23, issue 9, 2011 http://pharmtech.findpharma.com/pharmtech/Manuf acturing/Achieving-Aseptic-Drying-With-Spray- Drying-Technol/ArticleStandard/Article/detail/737355
21. P.T. Ingvarsson, M. Yang, H.M. Nielsen, J. Rantanen, C. Foged, Stabilization of liposomes during drying, Expert Opinion Drug Delivery, 8 (2011) 375-388
22. B. Tang, G. Cheng, J.C. Gu, C.H. Xu, Development of solid self-emulsifying drug delivery systems: preparation techniques and dosage forms, Drug Discovery Today, 13 (2008) 606-612
23. H. Takeuchi, S. Nagira, H. Yamamoto, Y. Kawashima, Solid dispersion particles of amorphous indomethacin with fine porous silica particles by using spray-drying method, Int J Pharm. 293, (2005) 155-164
24. A.A. Ambike, K.R. Mahadik, A. Paradkar, Spray-dried amorphous solid dispersions of simvastatin, a low tg drug: in vitro and in vivo evaluations, Pharmaceutical Research, 22 (2005) 990-998
25. C.G. Oster, T. Kissel, Comparative study of DNA encapsulation into PLGA microparticles using modified double emulsion methods and spray drying techniques. J Microencapsul. 22, (2005) 235-244
26. Y.F. Maa, S.J. Prestrelski, Biopharmaceutical powders: particle formation and formulation considerations, Curr Pharm Biotechno. 1, (2000) 283-302.
27. H. Schwartzbach. The possibilities and challenges of spray drying. Phamaceutical Technology Europe. Volume 22, Issue 5. 2010. http://pharmtech.findpharma.com/pharmtech/article/ articleDetail.jsp?id=667244&sk=&date=&&pageID=1
28. T.A.G. Langrish, New engineered particles from spray dryers: research needs in Spray Drying. Drying Technology, 25 (2007) 981–993