Regenerative medicine: a manufacturing overview
Posted: 27 August 2020 | Maciej Nakoniecznik (University College London) | No comments yet
The field of regenerative medicine – an area promising a revolution in 21st century healthcare – has been enabled by rapid scientific advancements in recent years. There is a growing need for a new generation of therapeutic products, made more severe by the global shortages of organs available for transplantations. However, before regenerative therapies can start playing a significant role in modern medicine, the industry must provide solutions to several key manufacturing challenges. Here, Maciej Nakoniecznik provides an overview of what the future holds for regenerative medicine.
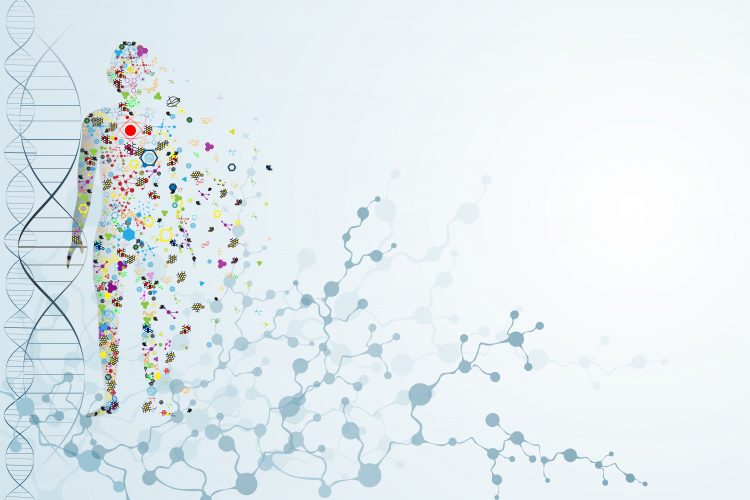
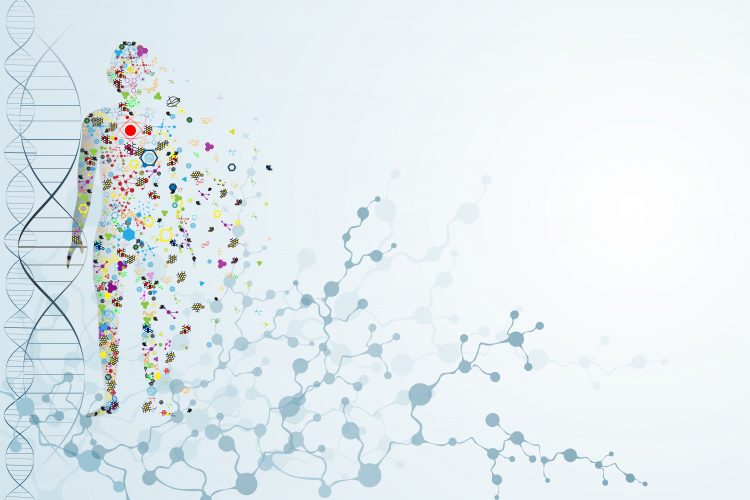
THERE ARE currently approximately 6,000 people awaiting lifesaving organ transplantations on the UK Transplant waiting list. In 2018/19, over 400 people died in the UK due to organ shortages.1 This figure is likely to be significantly higher this year, as safety restrictions introduced due to the COVID-19 pandemic saw the number of transplants performed between April and June plummet by over 50 percent compared to the same period last year.2 In the US, a steep growth in the number of transplants has been observed since 2013, with the number of patients on the transplant waiting list falling steadily during that period.3 However, over 112,000 people remained on the waiting list in September 2019, with more than 7,000 deaths attributed to organ shortages every year across the country.4
Most of the possible legislative changes aiming to increase the donor pool – such as the introduction of “death by donation” – have been rejected due to ethical concerns. A number of countries, including Spain, Belgium and most recently the UK, have switched to a system of “presumed consent” in order to increase the number of usable organs from deceased donors.5,6 The full effects of this legislation remain to be seen, but it is unlikely that its impact alone will be enough to overcome current organ shortages. The severity of the situation indicates that innovative steps will have to be taken; a promising potential solution is the emerging field of regenerative medicine and tissue engineering.
Definition of regenerative medicine
Manufacturing of regenerative medicine cellular products needs to be scaled out rather than scaled up to meet clinical demand”
Regenerative medicine is a rapidly evolving field that holds great promise for healthcare. It utilises a combination of cells and biological or synthetic scaffolds that are implanted into the patient, aiming to “replace or regenerate human cells, tissue or organs, to restore or establish normal function”.7 The definition clearly highlights the difference between regenerative medicine and current treatment methods, which largely focus on treating the effects of a given medical condition, rather than removing its underlying causes. The difference is well illustrated by the example of the current type 1 diabetes treatment, where the activity of pancreatic β cells is mimicked by insulin therapy. This strategy does not provide a cure for the disease, but rather aims to minimise its impact on patient health and quality of life. A potential treatment strategy applying the principles and techniques of regenerative medicine would aim to restore the normal insulin-secreting function of the β cells by replacing the depleted cell population, thus offering a cure for the condition, rather than merely a treatment of its symptoms.
Brief history and significance of regenerative medicine
Personalised medicine is a concept involving “tailoring the right therapeutic strategy for the right person at the right time” in place of the traditional “one-size-fits-all” approach.11 Personalised therapies are specifically adjusted for each patient using genomic and clinical data, increasing the effectiveness of the treatment and reducing the side effects compared to off-the-shelf drugs. Regenerative autologous cell therapies involving the uptake of cells from the patient’s body, in vitro modification and subsequent delivery back to the patient are likely to be the direction personalised medicine will take in the future. This approach has the potential to help solve many pressing problems of modern medicine, including the above-mentioned organ shortage; however, before it can make a wide-spread impact, a number of critical manufacturing challenges need to be addressed.
Key challenging areas
Quality control is challenging due to the complexity of the cells used in therapy compared to traditional drugs”
The challenges currently faced by the regenerative medicine manufacturing industry can be divided into three general categories: high manufacturing costs, production scale-up issues and regulatory hurdles. While some of these can be tackled by applying experience from pharmaceutical and biologics manufacturing, many are unique to this field and have not been encountered before.
In accordance with a key paradigm of personalised medicine – these therapeutic products are patient-specific and thus undergo a separate process in each instance – manufacturing of regenerative medicine cellular products needs to be scaled out rather than scaled up to meet clinical demand. The impact of economies of scale is therefore strongly reduced, resulting in elevated product prices. Process automation possibilities are currently limited, which leads to high reliance on human labour, further elevating the processing costs and increasing product variability. Transport logistics also remain a key cost-increasing factor, as cryopreservation, the current cell maintenance method of choice, may lead to significant product damage and requires specialist equipment for sustaining low temperatures during transport.12
Large-scale manufacturing of cell-based therapeutic products presents a unique challenge: the cells are the final product rather than a process impurity, unlike in the production of traditional biopharmaceuticals. For this reason, terminal sterilisation of the product cannot be performed; exogenous contamination must be avoided with other methods, such as reliance on closed systems and single-use technologies.13 Development of efficient upstream processing methods is also challenging. With autologous therapies, manufacturers should be able to run multiple processes in parallel within the same facility, which poses a high risk of cross-contamination. Development of novel processing platforms that combine the high throughput of other biotechnological industries with the precision and reliability of benchtop cell processing equipment is therefore key for further expansion of the field.
Summary
The need for development of scaleable, robust manufacturing processes for regenerative medicine products is imperative, as highlighted by the current worldwide organ donor shortages and limited capabilities of traditional medicine to treat the underlying causes of critical health conditions. If the idea of personalised medicine is to become a clinical reality, cell processing techniques must move beyond human-operated laboratory-based technologies and meet the emerging regulatory requirements. The main challenges currently faced by the industry involve high costs, problems with scale-up and regulatory ambiguity.
About the author
Maciej Nakoniecznik has recently been awarded a BSc degree in Bioprocessing of New Medicines at University College London, receiving numerous academic awards and is about to enrol on the MSc in Manufacture and Commercialisation of Stem Cell and Gene Therapies at the same institution. He has worked on a range of projects involving bioprocess design, centrifugation modelling and genomic data analysis, gaining substantial research experience.
References
- Organ donation and transplantation [Internet]. NHS Blood and Transplant. 2020 [cited 10 July 2020]. Available from: https://www.nhsbt.nhs.uk/what-we-do/transplantation-services/organdonation-and-transplantation/
- Donation and Transplantation Monthly Activity Report [Internet]. NHS Blood and Transplant; 2020 [cited 11 July 2020]. Available from: https://nhsbtdbe.blob.core.windows.net/umbracoassets-corp/19022/monthly-stats.pdf
- OPTN/SRTR 2018 Annual Data Report. American Journal of Transplantation. 2020;20(s1):1-568.
- National Survey of Organ Donation Attitudes and Practices, 2019 [Internet]. Rockville, Maryland: U.S. Department of Health and Public Services, Health Resources and Services Administration, Healthcare Systems Bureau; 2020 [cited 11 July 2020]. Available from: https://www.organdonor.gov/sites/default/files/about-dot/files/nsodap-organ-donationsurvey-2019.pdf
- A. Finding a solution to the organ shortage. Canadian Medical Association Journal. 2016;188(16):1182-1183.
- Opt-out organ donation: Max and Keira’s Bill passed into law [Internet]. GOV.UK. 2019 [cited 11 July 2020]. Available from: https://www.gov.uk/government/news/opt-out-organ-donationmax-and-keira-s-bill-passed-into-law
- Mason C, Dunnill P. A brief definition of regenerative medicine. Regenerative Medicine. 2008;3(1):1-5.
- Evans M, Kaufman M. Establishment in culture of pluripotential cells from mouse embryos. Nature. 1981;292(5819):154-156.
- Thomson J. Embryonic Stem Cell Lines Derived from Human Blastocysts. Science. 1998;282(5391):1145-1147.
- Takahashi K, Tanabe K, Ohnuki M, Narita M, Ichisaka T, Tomoda K et al. Induction of Pluripotent Stem Cells from Adult Human Fibroblasts by Defined Factors. Cell. 2007;131(5):861-872.
- Official Journal of the European Union, C 421 [Internet]. European Commission; 2015 [cited 12 July 2020]. Available from: https://eur-lex.europa.eu/legal-content/EN/TXT/PDF/?uri=OJ:C:2015:421:FULL&from=EN
- Cohen P, Hunsberger J, Atala A. Regenerative Medicine Manufacturing – Challenges and Opportunities. In: Atala A, Lanza R, Mikos A, Nerem R, ed. by. Principles of Regenerative Medicine. 3rd ed. Elsevier; 2019. p. 1367-1377.
- Pattasseril J, Varadaraju H, Rowley J. Downstream Technology Landscape for Large-Scale Therapeutic Cell Processing. BioProcess International. 2013.
- Martin I, Simmons P, Williams D. Manufacturing Challenges in Regenerative Medicine. Science Translational Medicine. 2014;6(232):232fs16-232fs16.
Issue
Related topics
Biopharmaceuticals, Bioprocessing, Bioproduction, Personalised medicine, Regenerative Medicine, Stem Cells