Evolution of drug metabolism and pharmacokinetics in drug discovery and development
Posted: 10 July 2012 | | No comments yet
Drug Metabolism and Pharmacokinetics (DMPK) is a scientific discipline once primarily associated with safety evaluation in drug development that has, in the last two decades, become a core discipline within drug discovery, development and even post-marketing. Approximately 20 years ago, sub-optimal DMPK properties were recognised as a contributor to the failure of potential new therapies in early clinical trials and this precipitated the realignment of DMPK within discovery to assist in selecting optimal drug candidates to enter clinical trials. In addition to adequate potency against the target protein and an acceptable safety profile, a balance of optimised PK parameters and minimised drug-drug interaction (DDI) potential maximises the chance of a candidate drug becoming a successful therapy.
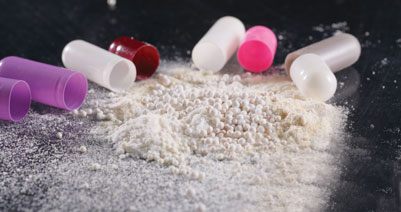
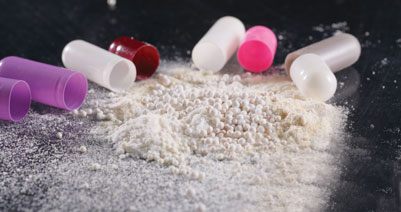
Drug Metabolism and Pharmacokinetics (DMPK) is a scientific discipline once primarily associated with safety evaluation in drug development that has, in the last two decades, become a core discipline within drug discovery, development and even post-marketing. Approximately 20 years ago, sub-optimal DMPK properties were recognised as a contributor to the failure of potential new therapies in early clinical trials and this precipitated the realignment of DMPK within discovery to assist in selecting optimal drug candidates to enter clinical trials. In addition to adequate potency against the target protein and an acceptable safety profile, a balance of optimised PK parameters and minimised drug-drug interaction (DDI) potential maximises the chance of a candidate drug becoming a successful therapy.
The front loading of DMPK to drug discovery can be attributed to a widely used but extremely misleading statistic from an analysis of compounds in clinical development between 1964-1985 in UK companies, where approximately 40 percent of drug attrition was attributed to sub-optimal PK properties. However, if the anti-infective class of drugs was removed from this analysis, the percentage lowers to less than 10 percent1-3. Moreover, a recent analysis published in 2005 puts the attrition due to poor PK properties at a similar rate of 10 per cent4. So, despite frequent suggestions to the contrary, there is little actual evidence of a transformative reduction of attrition due to optimising PK properties over the last two decades.
However, that is not denying the tremendous progress DMPK scientists have made in understanding absorption, distribution, metabolism and excretion (ADME) processes, contributing to enhanced drug design and the avoidance of DDIs, especially when set against a background of rising commercial, societal, regulatory and budgetary pressures. Reducing attrition is the current focus for R&D and DMPK has a key role to play, not only in discovering and developing drugs with sufficient oral bioavailability, adequate half-lives and minimising potential for DDI liabilities, but to contribute via reducing attrition for lack of efficacy and poor safety profiles.
The central role of DMPK in drug discovery is to contribute to the optimisation of compounds for man by balancing the properties associated with drug gastrointestinal absorption (for orally delivered therapies), distribution, clearance, elimination and DDI potential as rapidly and cost effectively as possible. This can be achieved from a consideration of physicochemical properties and data obtained from in vitro assays with human-derived material and in vivo animal studies. There are several approaches currently used to predict human PK including allometric scaling and in vitro-in vivo extrapolation, albeit some of the methodologies have common inputs. Allometry relates in vivo derived parameters across species as a power function to differences in body weight. However, the predictive capability of allometry is limited for low clearance compounds, a requirement for oral drug candidates, as it does not account for species differences in metabolism. Determining metabolic clearance from human in vitro tissue preparations such as hepatocytes and scaling to the whole body has been successfully used for several years.
DMPK science is evolving from making discrete measurements in in vitro milieu and in vivo experiments in preclinical animals to develop the wherewithal to predict PK and DDI potential in man. Rapid feedback to medicinal chemistry led design teams providing estimates of human PK and predicted therapeutic dose are pivotal to the efficient optimisation of lead compounds into candidate drugs. Early dose predictions provide a means of integrating biological and PK data and thus provide a holistic assessment of overall compound quality. The Design Make Test Analyse (DMTA) cycle is the multi-disciplinary engine of lead optimisation and drug discovery screening cascades. The most efficient DMTA cycles are based on screening cascades appropriate for projects typically starting with a panel of assays in ‘Wave I’ determining potency against the target, physicochemical properties and basic in vitro data such as metabolic stability and cytochrome P450 inhibition assessment. Thus, subsequent in vivo studies aimed at establishing, and if necessary optimising, in vivo efficacy can be performed with better quality compounds. There are different levels of PK prediction and compound design inputs of increasing complexity, ranging from purely in silico methods via extensive in vitro and in vivo data to physiological based pharmacokinetic models, which characterise drug distribution to specific organs in terms of physiologically relevant variables.
Arguably, over the next decade and beyond in our discipline, it is in silico DMPK approaches that can most significantly impact the cost effectiveness, speed and design quality of drug discovery projects. Conducting ‘Wave 0’ virtual screens of sufficiently high predictive capability before chemical synthesis is the single most cost effective contribution DMPK can make to improving drug design. However, to be maximally impactful, in silico models need to improve current levels of predictivity, become more mechanistic and importantly provide design teams with the ability to optimise multiple properties simultaneously.
A real success that DMPK can claim in the last decade or so is establishing the in vitro assays and predictive capability to minimise the potential of cytochrome P450 mediated DDI with candidate drugs as ‘perpetrators’ or ‘victims’. The incorporation of cytochrome P450 inhibition and reaction phenotyping data from simple and rapid screens can identify the clinical consequences for candidate drugs and provide the basis for DDI avoidance strategies. Approaches based on such in vitro assays and in vivo predictions appear relatively successful at estimating the degree of such clinical interactions and can be readily incorporated into early drug discovery. Sophisticated dynamic approaches now exist such as the commercially available software, Simcyp (www.simcyp.com) which, whilst still based on in vitro data available in drug discovery, has become a useful prediction and simulation tool for use within all phases and in the rationale and design of clinical DDI studies via simulating DDI and PK outcomes in virtual clinical populations. Bases for variability in drug responses in populations have been known for many years. Genetic polymorphisms of drug metabolising enzymes have long been identified as responsible for differing drug exposures in individuals, but the relatively recent advances in molecular biology and genome mapping has led to a still developing understanding of the role of pharmacogenomics in inter-individual and inter-ethnicity differences in drug disposition, efficacy and toxicity. Identifying genetic factors that determine a patient’s response to a drug should lead to the development and use of personalised, safer and more efficacious therapies.
After identification of the candidate drug, the role of DMPK is to increase knowledge of the ADME and PK properties in relevant animal species and man in support of clinical drug development. A primary objective throughout this phase is to provide a continuous assessment of the dose-exposure-effect relationship in non-clinical and clinical studies and contribute to study conduct, timing and design. With this goal in mind, the DMPK capability works as part of a multi-disciplinary team to optimise the benefit-risk ratio along the development path. In recent years, more detailed elucidation of metabolic profiles in pre-clinical and clinical studies has been required. Significant effort is now dedicated in drug development to detect, identify and if possible quantify stable metabolites circulating in man that are pharmacologically active or not represented by preclinical safety species and chemically reactive metabolites that, via downstream events, result in increased toxicological risk.
The last decade or so has seen the increasing realisation and understanding of the importance of membrane transporters in drug disposition, safety and efficacy. Transporters have a role in the oral absorption, distribution and excretion of drugs and there are now several well established, clinically important, transporter mediated DDIs. Transporters important to drug development are located in the epithelia of intestine, kidney and liver and in the endothelium of the blood brain barrier and are from two major families, ATP-binding cassette (ABC) and solute carrier (SLC). Recently, an international consortium outlined recommendations on the most important transporters for drug development and the appropriate conduct of in vitro and in vivo studies in support of clinical programs5. A key area of current and future research is to further our knowledge of the interplay between hepatic transporters and metabolic processes and hepatic and renal transporters implicated in biliary and urinary clearance processes respectively and to develop the ability to design out associated liabilities in candidate drugs. Moreover, the role of DMPK in predicting and interpreting hepatotoxicity has increased recently due to technological advances that have improved analysis of transporter interactions and liver/biliary tract exposure and the identification of potential reactive metabolites.
In recent years, the number of large molecule biologics drug candidates, including peptides and monoclonal antibodies, has increased dramatically due to advances in biotechnology and an apparently lower attrition rate compared to traditional small molecule drug candidates6. Thus, effort is now applied to determining the ADME properties of large molecule drug candidates in support of clinical development primarily applied in establishing a pharmacokinetic-pharmacodynamic (PKPD) relationship and for establishing clinical dosing regimen. Although fundamental PK principles apply equally to large and small molecule drugs, the actual properties of large molecule drugs are usually so significantly different from their small molecule counterparts, the focus and approaches used can also be somewhat divergent. Clearly, this is an important current and future area to develop our DMPK knowledge and apply innovative, value adding contributions to these programs.
Reducing attrition via increasing the quality of both candidate drugs and non-clinical / clinical studies has been a goal for DMPK and other pharmaceutical scientists for years yet recently this has needed to be achieved under more constrained R&D budgets. Thus, the strategy, widely implemented in the 1990s of frontloading more studies earlier in the drug value chain and screening more compounds in more projects may not now be achievable or indeed appropriate. Complex risk judgements now need to be made in order to maximise the likelihood of success which necessitates higher quality in cerebro input from DMPK scientists than development of any single assay or technology. With that in mind, in active collaboration with academia, we in the pharmaceutical industry need to train, recruit and retain innovative and talented DMPK scientists as a priority investment for our science and contribution towards successful drug discovery and development in the future.
Predictive DMPK methodologies do not offer a panacea for successful PK and dose prediction. A successful PK prediction can be reasonably characterised as estimating the key parameters within twofold. However, successful predictions of individual PK parameters may not equate to a successful prediction of clinical dose and associated exposure7. Nonetheless, these methodologies, which are continually undergoing refinement, should make a positive and important contribution to improving the unsustainably high attrition rate in drug development and lead to the more rapid and economical provision of effective and safe therapies. DMPK has an important and unique role as a scientific discipline at the interface of multiple disciplines including medicinal and bioanalytical chemistry, bio – science, personalised healthcare, safety assessment, pharmaceutical development, clinical and regulatory affairs. The last two decades have shown much progress in advancing DMPK as a science and developing the role and contribution of the discipline to drug discovery and development. However, in recent years, the lack of identifiable druggable targets combined with the complexity of in vivo biological systems and the increasingly challenging hurdles from regulators and payers have resulted in stubbornly high drug attrition rates, so the paradigm of bringing effective, safe medicines that make a meaningful difference to patients via an affordable and sustainable means is proving challenging to deliver. Over the next period of time, DMPK scientists in the pharmaceutical industry and partner organisa – tions need to focus all their intellect and energies in contributing to this overall goal.
References
- Kubinyi, H. Nat Rev Drug Discov (2003) 2: 665-668
- Kennedy, T. Drug Discov Today (1997) 2: 436-444
- Prentis RA et al., Br J Clin Pharmacol (1988) 25:387-396
- Schuster D et al., Curr Pharm Des (2005) 11:3545-3559
- Giacomini KM et al., Nat Rev Drug Discov (2010) 9: 215-236
- Lin JH. Curr Drug Metab (2009) 10: 661-691
- McGinnity DF et al., Curr Drug Metab (2007) 8: 463-479
About the author
Dermot McGinnity graduated in Biochemistry and completed a PhD on cytochrome c peroxidases at the University of Edinburgh. Dermot trained as a post-doc with Professor Jud Coon at the University of Michigan researching the catalytic mechanisms of cytochrome P450. In 1997, he joined Discovery DMPK at AstraZeneca Charnwood in the UK, ultimately reaching the position of Department Director. Dermot was part of the team that discovered the recently launched BRILINTA and is currently Global Director of Screening & Profiling, DMPK in AstraZeneca Mölndal, Sweden. Dermot’s research interests include enhancing the predictive capability of human pharmacokinetics and clinical drug-drug interactions.