Meeting the challenge of cost-effective OTC drug manufacture with powder testing
Posted: 20 August 2021 | Jamie Clayton | No comments yet
Sharper manufacturing practice is often essential to rigorously optimise production economics and compete effectively in the over-the counter (OTC) marketplace. This article describes the application of powder testing to improve the manufacturing efficiency of OTC products detailing work by Pfizer and GlaxoSmithKline (GSK), companies with extensive OTC portfolios.
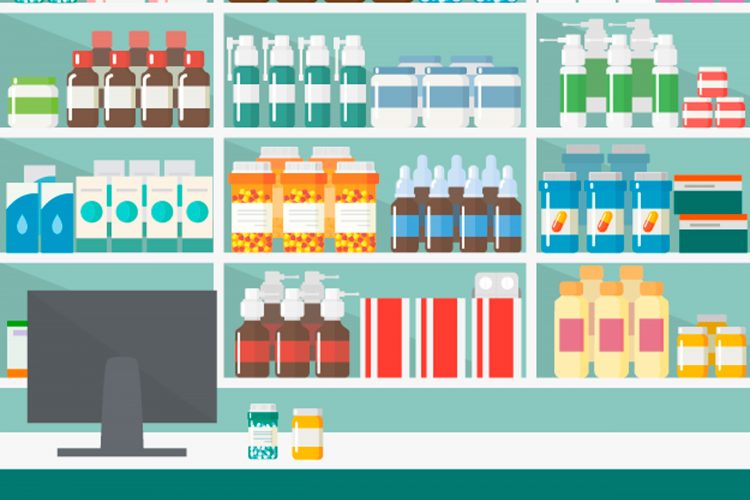
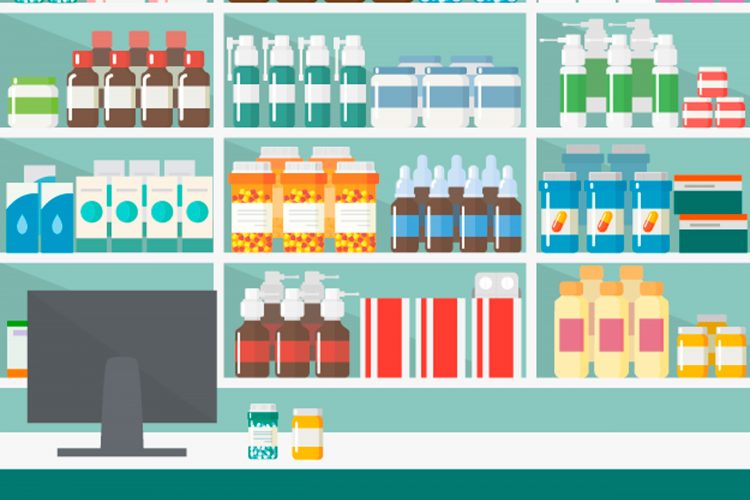
Across Europe sales of over-the counter (OTC) drug products have increased during the pandemic, with France and the UK recording the highest levels of growth, 7.1 and 5.7 percent respectively.1 Longer term trends show OTC use rising steadily across the globe.2 The OTC market is consequently an increasingly attractive proposition for pharmaceutical manufacturers and an ‘Rx (prescription) to OTC switch’ is a relatively common strategy, post patent expiry, that can help to maintain profitability in the face of generic competition, as well as extending brand awareness.
Over 700 Rx products have been granted OTC status by the US Food and Drug Administration (FDA) over the last 30 years.3 However, the OTC marketplace is cost-sensitive, with the growth in private-label products (products sold under the name of the retailer rather than the manufacturer) an important factor with respect to downward pressure on price.3 Here, we illustrate using case study data, how powder testing can help manufacturers rise to the challenge of more efficient OTC product manufacture and compete effectively.
Working towards more efficient tableting
Faced with rising demand for an OTC oral solid dosage product, Pfizer turned to powder testing to identify a strategy for alleviating capacity constraints associated with a high shear wet granulation (HSWG) process and with subsequent tableting. A study was carried out to tackle two aspects of design space definition to provide a sound foundation for improvement. In a first step, correlations were established between HSWG processing parameters and the properties of the resulting granules and, in a second, granule properties were correlated with critical quality attributes (CQAs) of the finished tablets.
Table 1 shows the results of a pilot scale design of experiments (DoE) study exploring the impact of HSWG process parameters on wet granule flow properties; processing parameter values were derived from full-scale manufacturing plant operation. HSWG involves introducing water into a fine powder and applying shear to promote the formation of larger granules and is used routinely to enhance the flowability and compressibility profile of tableting blends and to reduce segregation. Granule properties are controlled by manipulating various factors: the solid to water addition ratio; impeller speed; kneading time; and binder temperature. The data show how these variables impact the flowability of granules immediately exiting the granulator, as quantified by Basic Flowability Energy (BFE), a dynamic powder flow property.4
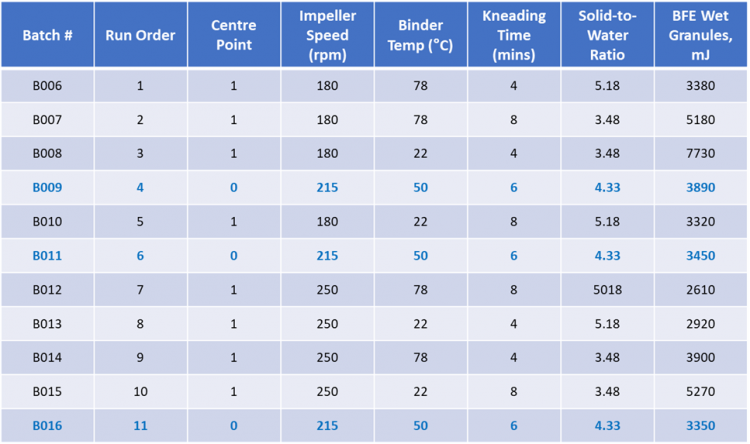
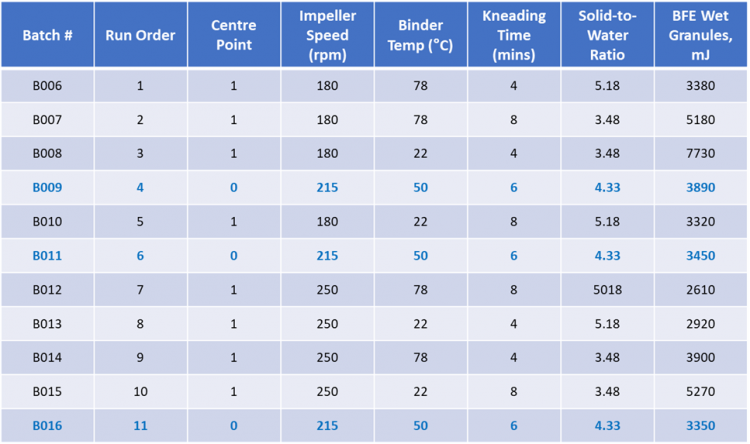
Table 1: Data from a pilot scale DoE study of HSWG shows how process parameters can be manipulated to generate granules with a target flowability, as quantified by BFE.
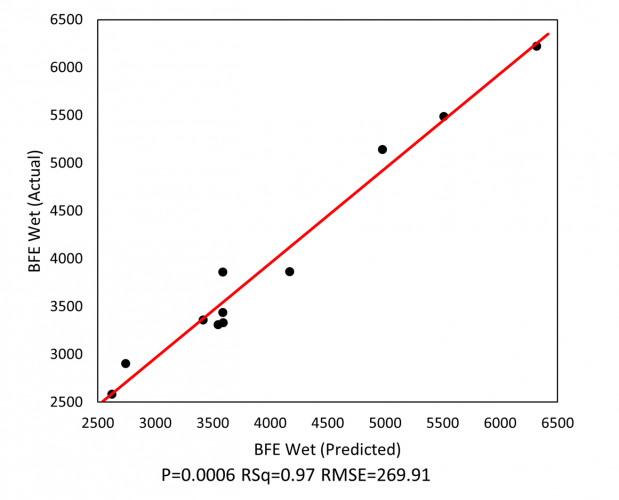
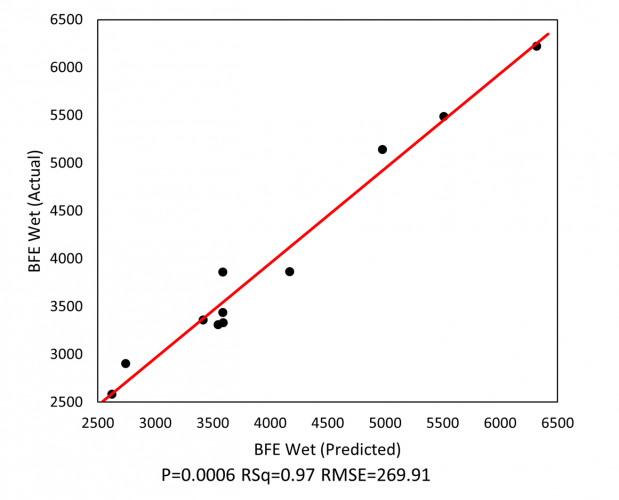
Figure 1: Granule flowability, as quantified by BFE, can be reliably predicted from values of HSWG processing parameters.
Analysis indicates that the BFE of the wet granules is a function of solid-to-water ratio, impeller speed and binder temperature, and can be successfully predicted from HSWG process parameters (see Figure 1). The ability to apply dynamic testing to the wet granules enables early process evaluation and, by extension, more responsive process control.
![Table 2: Once granules have been dried a wider range of properties can be measured, including permeability (ΔP), additional dynamic parameters (aerated energy) and shear properties (cohesion and flow function [FF]).](https://www.europeanpharmaceuticalreview.com/wp-content/uploads/OTC-Table2-750x445.png)
![Table 2: Once granules have been dried a wider range of properties can be measured, including permeability (ΔP), additional dynamic parameters (aerated energy) and shear properties (cohesion and flow function [FF]).](https://www.europeanpharmaceuticalreview.com/wp-content/uploads/OTC-Table2-750x445.png)
Table 2: Once granules have been dried a wider range of properties can be measured, including permeability (ΔP), additional dynamic parameters (aerated energy) and shear properties (cohesion and flow function [FF]).
With dry granules it is feasible to measure bulk powder properties such as permeability, to apply a wider range of dynamic tests including aeration testing and to determine shear properties such as cohesion and flow function (FF). Analysis of these results (Table 2) highlights the strong influence of solid-to-liquid ratio and demonstrates the feasibility of predicting any of the measured properties from HSWG processing parameters, though the strongest correlations observed were for BFE and permeability. However, given that the goal is to improve tableting, then it is those properties that define performance in the tablet press that are of most relevance for control of the HSWG step. A second series of experiments was carried out to identify these properties.
Tablets were made with granules from each of the batches. The tablet press was run at 35rpm to accommodate all batches, with fill depth and compression pressure changed to achieve tablets of comparable weight and thickness, despite differences in flowability. An adjusted measure of hardness was determined for subsequent analysis of the data, to compensate for these differences in tableting parameters. This adjusted measure of hardness was found to correlate strongly with the BFE of wet (see Figure 2) and dry granules (data not shown).
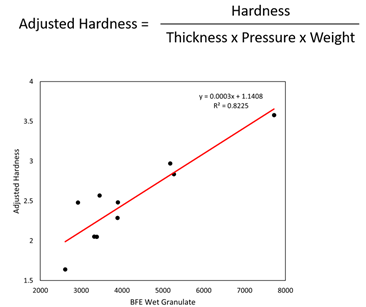
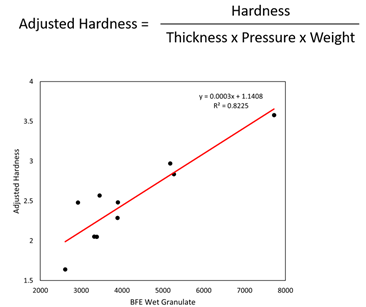
Figure 2: Tablet hardness (adjusted), a CQA, correlates with the BFE of wet granules, illustrating the benefit of controlling the HSWG to the produce granules of defined flowability.
This finding is consistent with previous studies5,6 and underlines the utility of BFE measurements for HSWG control. With such correlations in place, it becomes possible to target a specific BFE to produce tablets of the required quality in the most efficient way. For example, reducing kneading time will help to increase throughput and may be feasible if compensatory changes are made to other processing parameters such as solid-to-liquid ratio or impeller speed. As such, powder testing can be applied here to help Pfizer to establish the understanding required to shift towards more efficient manufacturing and attain the goal of higher throughput.
Troubleshooting solid dosage manufacturing processes
For GlaxoSmithKline (GSK), a primary focus for the application of powder testing was to improve supply chain management and, more specifically, to optimise the choice between alternative, commercially available supplies. A major problem with legacy processes is that performance can deteriorate gradually over time as alternative raw materials prove progressively harder to process, without any real understanding of why.
Powder testing was carried out to define specifications for a legacy tableting line exhibiting this problem, with a throughput of around 50 percent of historical levels. These studies identified compressibility and flowability, as quantified via dynamic testing, as critical parameters for the tableting blends and the results were used to identify raw materials that could restore performance. In situations such as this, a powder tester can easily deliver savings of between 50 to 100 times its cost,7 underlining the real impact that relevant testing can have.
GSK has also used powder testing to refine the raw material selection process for OTC respiratory powders produced by dry granulation (compaction). Here compressibility, which quantifies change in bulk density (or volume) as a function of consolidation pressure (see Figure 3) is highly relevant and optimal process settings have been determined to process feeds of varying compressibility since alternative supplies that notionally meet the same specification differ considerably in this regard.
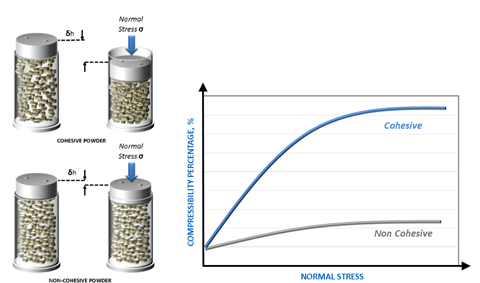
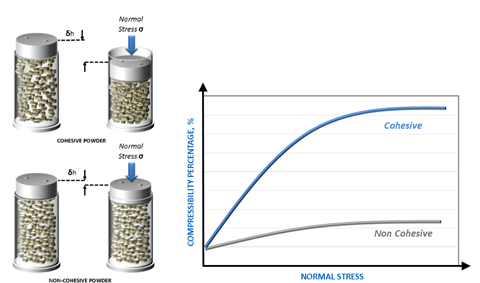
Figure 3: Compressibility quantifies the response of a powder to applied normal stress and is particularly relevant to compaction and tableting processes.
For example, testing of alternative supplies of an active ingredient revealed that compressibility, which was typically five percent, could be as high as 25 percent in some instances. Supplies with a compressibility of 10 percent were successfully compacted using a higher compaction pressure, but a supply with 25 percent compressibility was simply not processable. For this powder, the compaction force required to produce a stable ribbon was higher than the equipment could apply; the powder lay outside the boundaries of an acceptable specification for the process.
This is an interesting and valuable finding. All these supplies met the same market specification but proved different with respect to process performance. Imposing a more stringent specification within the marketplace invites higher costs so, choosing between supplies on the basis of in-house testing is more cost-efficient. This example demonstrates how powder testing can be implemented, allowing the efficient rejection of a supply that will cause a process failure.
Beyond raw material selection, GSK has also successfully used powder testing for other aspects of process optimisation, for example, to eliminate an issue with segregation in a hopper. In this instance, the segregation necessitated wasting material from every batch, due to a risk of super- or sub-potency at low powder levels. Using shear testing in combination with integral hopper design software, critical dimensions for a suitable hopper design – hopper half angle and outlet size – were determined.8 This in-house exercise led to the specification of a new hopper 50 percent larger in volume than the original, with different critical dimensions, that empties more quickly and exhibits no product segregation. Video footage shows that the new hopper maintains a desirable mass flow regime, eliminating the funnel flow associated with the original design, and the associated rolling segregation. Overall, the exercise resulted in an eight percent material saving.7
In conclusion
These example studies highlight the merit of powder testing and its broad utility in the rigorous optimisation of OTC manufacturing. Relevant powder testing can play an important role in realising the high manufacturing efficiency and competitive production economics needed to thrive in this marketplace, as experience from two global leaders illustrates.
However, this work simultaneously underlines two other vital points. Firstly, the importance of multi-faceted powder characterisation, the criticality of being able to measure dynamic, bulk or shear properties, depending on the application of interest; all three types of properties are referenced here. Secondly, the value of having those capabilities in-house to ensure that powder testing can be applied as and when required.
In all examples the powder testing used Freeman Technology’s FT4 Powder Rheometer®.
About the author
Jamie Clayton is Operations Director at powder characterisation company Freeman Technology, based at the company’s headquarters in Tewkesbury, UK. He graduated from University of Sheffield with a degree in Control Engineering and is responsible for overall management of company activities, including the R&D, production, sales and customer support teams. During his time with the company, Jamie has worked as a mentor with several academic groups and is an active member of ASTM F42. He is also a regular contributor to conferences and workshops on the topic of powder rheology and works closely with clients on the application of the company’s technology.
References
- Monitoring the Impact of COVID 19 on the Pharmaceutical Market EU4 & UK [Internet]. IQVIA; 2021 [cited August 2021]. Available from: https://www.iqvia.com/library/white-papers/monitoring-the-impact-of-covid-19-on-the-pharmaceutical-market-eu5
- OVER THE COUNTER DRUGS MARKET – GROWTH, TRENDS, COVID-19 IMPACT, AND FORECASTS (2021 – 2026) [Internet]. Mordor Intelligence; 2021. Available from: https://www.mordorintelligence.com/industry-reports/global-over-the-counter-otc-drugs-market-industry
- Rein A. How Trends in Over-The-Counter Products are Shaping the Market – Lubrizol [Internet]. Lubrizol.com. 2021 [cited August 2021]. Available from: https://www.lubrizol.com/Health/Blog/2020/04/How-Trends-in-Over-The-Counter-Products-are-Shaping-the-Market
- Freeman R. Measuring the flow properties of consolidated, conditioned and aerated powders — A comparative study using a powder rheometer and a rotational shear cell. Powder Technology. 2007;174(1-2):25-33.
- Freeman, T. (2014) ‘In Pursuit of Wet Granulation ’ Pharmaceutical Manufacturing. Available to view at: http://www.pharmamanufacturing.com/articles/2014/in-pursuit-of-wet-granulation-optimization/
- Cassidy OE, Thomas WI. Characterisation of wet masses using a powder rheometer Poster Session 1 – Pharmaceutics. Journal of Pharmacy and Pharmacology, 54 (2002) 25-46.
- Mollner T. Why powder testing is a powerful tool for healthcare. European Pharmaceutical Manufacturer. 30 January 2019. Available at: https://www.epmmagazine.com/pharma-manufacturing-news/why-powder-testing-is-a-powerful-tool-for-healthcare/
- Freeman T. Modern Tools for Hopper Design. Available at: https://www.freemantech.co.uk/news/modern-tools-for-hopper-design
Related topics
Active Pharmaceutical Ingredient (API), Analytical techniques, Big Pharma, Drug Manufacturing, Research & Development (R&D), Technology, Therapeutics
Related organisations
GlaxoSmithKline (GSK), Pfizer, US Food and Drug Administration (FDA)