Paving the way for anti-Abeta active immunotherapy
Posted: 22 September 2023 | Dr Andrea Pfeifer (AC Immune), Dr Marie Kosco-Vilbois (AC Immune), Dr Marija Vukicevic (AC Immune), Dr Yves Kremer (AC Immune) | No comments yet
Recent breakthroughs in anti-Abeta immunotherapy for Alzheimer’s have shown disease-modifying potential, with vaccination emerging as a promising approach, as AC Immune’s Marija Vukicevic, Yves Kremer, Marie Kosco-Vilbois and Andrea Pfeifer explain.
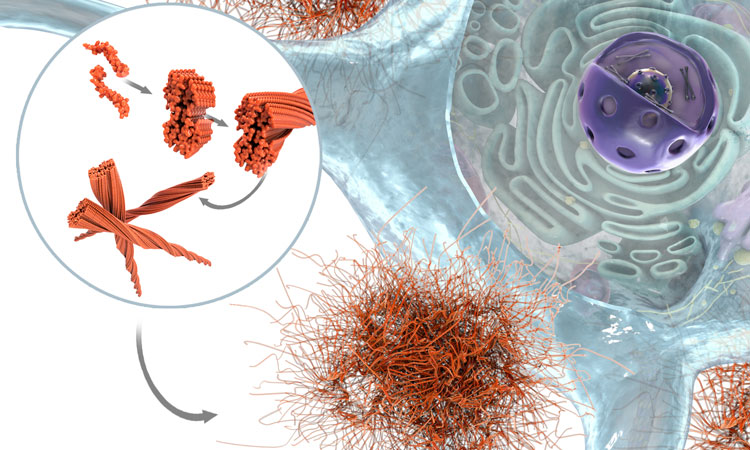
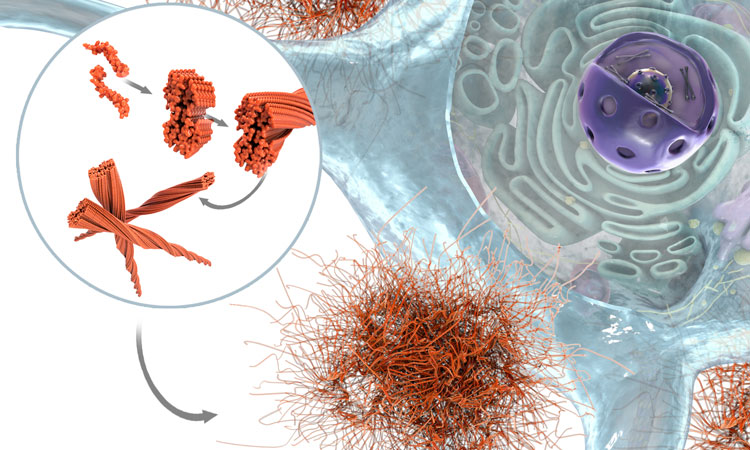
From Abeta peptides to toxic oligomers and plaque. [Credit AC Immune]
After decades of research efforts, targeting extracellular amyloid beta (Abeta) has recently been shown to have a disease-modifying effect with anti-Abeta monoclonal antibodies (mAb) making breakthroughs in Phase III trials and clinically validating the amyloid hypothesis. Targeting toxic species of Abeta, such as multimers (with aducanumab and lecanemab) or pyroglutamate Abeta3-42 (with donanemab), induced significant removal of Abeta plaque load in the brains of Alzheimer’s patients, leading to the delay in cognitive decline.
Limitations of monoclonal antibody therapies
Regulatory approvals from the US Food and Drug Administration (FDA) for aducanumab and lecanemab – and likely very soon for donanemab also – opened a route for different therapeutic modalities and other relevant disease targets, such as tau. Treatment with the mAbs delayed cognitive decline by 2.6 months (aducanumab) and the more meaningful amounts of 5.1 months for lecanemab and 5.5 months for donanemab.1
However, infusions of different doses of mAbs resulted in dose-dependent amyloid-related imaging abnormalities vasogenic edema (ARIA-E) of varying degrees (in up to 41 percent, 26.7 percent and 14.3 percent of patients injected with, respectively, aducanumab, donanemab and lecanemab). These ARIA-E effects are attributed to very high doses of mAbs leading to peak levels as measured in plasma and cerebrospinal fluid (CSF). These peaks are rapidly followed by a decrease to the minimal (trough) levels, after which the mAbs are re-infused, either monthly (donanemab) or every second week (lecanemab), in order to maintain efficacy.
These mAb treatments are further complicated by anti-drug antibody (ADA) responses in patients. For lecanemab this appears to be a relatively low-level response with little impact on treatment.2 However, for donanemab more than 90 percent of patients developed anti-drug antibodies three months after the first dose and these persisted for three months after the last dose.3 This high level of ADA induction may explain the reductions in treatment effects, despite high injected doses of the antibody.
While new positive clinical data with anti-Abeta mAbs signal tremendous advancement and proof‑of-concept for the whole field, it is believed that the observed effects could still be improved and optimised in terms of greater clinical benefits, lower side effects and enhanced treatment convenience.
Active anti-Abeta immunotherapies
In the 2000s, the concept of active immunotherapy targeting Abeta in Alzheimer’s patients was assessed with the ELAN vaccine (AN1792), consisting of full-length aggregated Abeta 1-42 peptide mixed with the QS21 adjuvant. The vaccination of patients with AN1792 led to the activation of their immune systems, which started producing endogenous anti-Abeta antibodies. In 19.7 percent of immunised patients who developed high anti-Abeta antibody titers, the lowering of brain Abeta was measured and hints of clinical efficacy were observed.4
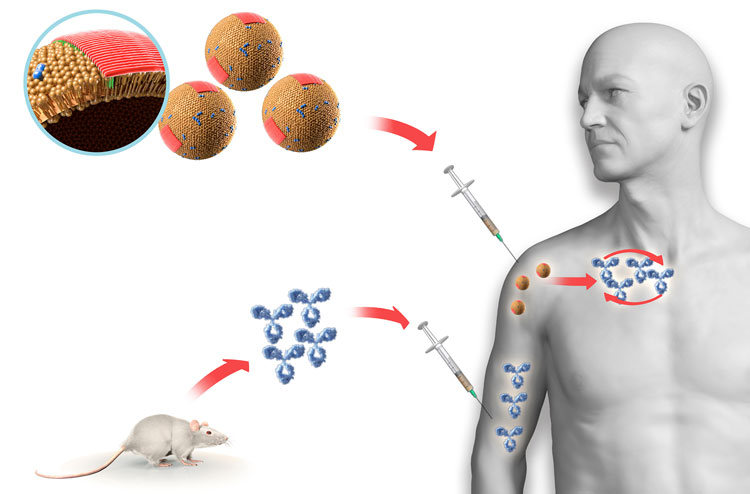
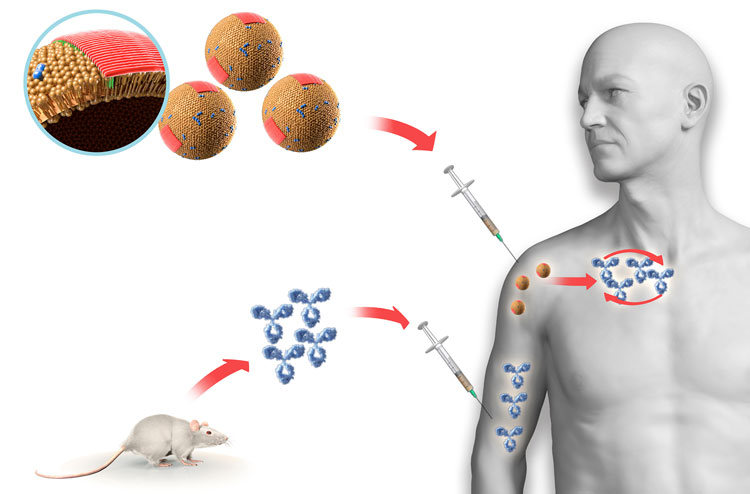
Vaccines compared to passive immunotherapy [Credit AC Immune]
However, the clinical trial was terminated early, as six percent of patients developed meningoencephalitis, likely due to the infiltration of Abeta-specific T cells in the brain producing inflammation.5 The lesson learned from this trial was that vaccines containing Abeta T-cell epitopes should be avoided. The next generation of active immunotherapies was based on Abeta peptides with the capacity to activate only Abeta-specific B cells, but not T cells. This was achieved by using the N-terminal part of Abeta that is devoid of dominant Abeta T-cell epitopes.6
The proof-of-concept from the mAbs shows the relevance of immunologically targeting extracellular toxic Abeta species but the aforementioned drawbacks of this approach must be considered. Therefore, active immunotherapy to trigger the immune system to produce sustained levels of endogenous antibodies has now become far more attractive.
There are multiple advantages of active immunotherapy through vaccination (see Table 1):
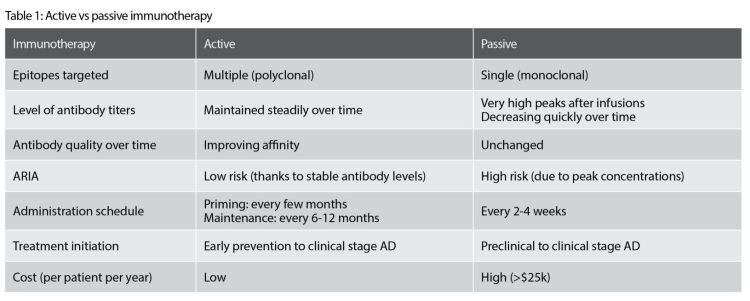
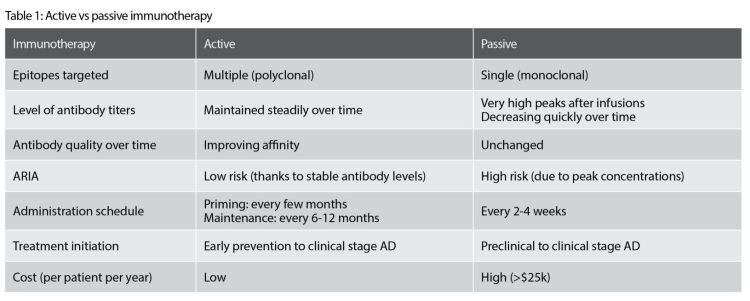
*amyloid-related imaging abnormalities
- The human immune system is activated to produce a long-lasting polyclonal antibody response that has a broader epitope coverage (eg, specific for multiple toxic species) than the mAbs, which each target a single epitope.
- Once the initial polyclonal response is induced, it can be restored and maintained with occasional immunisations (eg, every six to 12 months). Conversely, mAb infusions lead to high peak levels in the circulation, which subsequently decrease rapidly, necessitating repeat injections every two to four weeks.
- Antibody responses induced with active immunotherapy are polyclonal and self‑optimised in their specificity towards the pathological target relevant for human pathology, through the process of affinity maturation.
- The constant and steadily maintained antibody levels of the polyclonal antibody response carry a lower risk of inducing ARIA-E, as compared to the rapid extreme fluctuations in antibody levels experienced with mAb therapies.
- Patient convenience is far greater with a vaccine administration schedule requiring only a limited number of injections each year, as compared to burdensome intravenous infusions every few weeks for mAbs.
- Active immunotherapy through vaccination has a far lower cost than mAb-based therapies, representing an important decrease of economic burden for healthcare systems at a global scale.
Anti-Abeta active immunotherapies currently in clinical development
Currently, there are several anti-Abeta active immunotherapies in clinical development (Table 2).
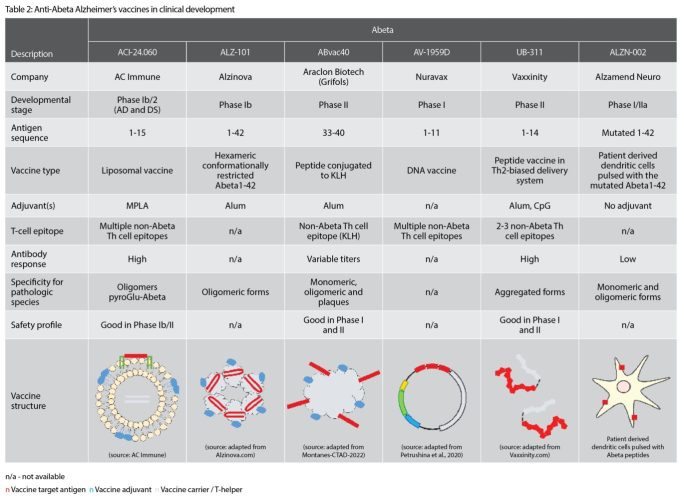
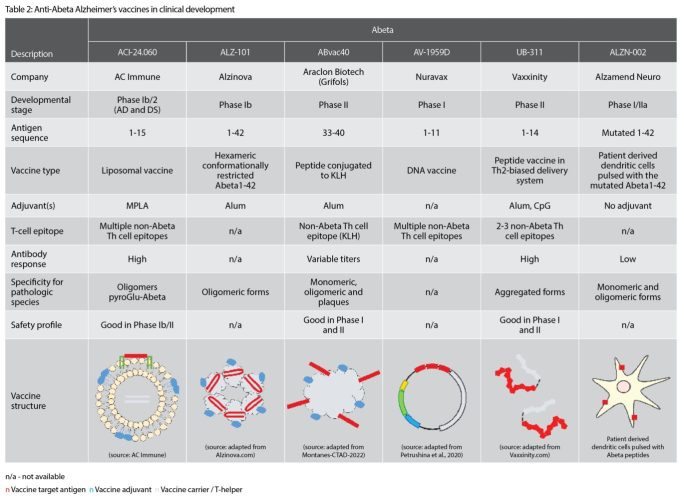
AC Immune’s vaccine candidate, ACI‑24.060, is a liposomal active immunotherapy, presenting the Abeta 1-15 peptide in a unique, conformationally‑restricted manner such that the induced antibody response shows very high specificity towards the pathological Abeta species such as oligomers and pyroglutamate Abeta.7 It is currently being evaluated in a Phase Ib/IIa clinical study, demonstrating to date a good safety profile. ALZ‑101 (Alzinova) immunotherapy, currently in a Phase IIb trial, contains hexameric, conformationally-restricted Abeta1-42 peptides mixed with alum adjuvant, leading to the production of antibodies preferentially binding to the oligomeric forms of Abeta.
ABvac40 (Araclon Biotech) is a KLH-conjugate bound to the C-terminal region of Abeta (33-40) and induces antibodies binding to different forms of Abeta, including monomers, oligomers and plaques. While favourable safety has been reported up to the Phase II, the relevance of targeting the C-terminal portion of Abeta still needs to be confirmed. In addition, a KLH protein carrier cannot be easily used in marketed products due to the significant variability of this natural material.
AV-1959D from Nuravax is a DNA-based therapeutic, containing Abeta1-11 sequence bound to multiple T-cell helper epitopes.
UB-311 from Vaxxinity is composed of two copies of Abeta1-14 peptide, each bound to a different T-cell helper peptide and further mixed with alum and CpG adjuvants. UB-311 has demonstrated favourable safety in Phase I and II studies and suggested in Phase II a potential improvement of cognitive function.8
[Editor’s note: Since this article was written in June 2023, results from a randomised, double-blind, placebo-controlled, Phase IIa study of UB-311 have been published in the Lancet. The Phase IIa study met its primary objectives of demonstrating safety, tolerability, and immunogenicity.]
Taken together, the accumulating data for vaccines demonstrates general favourable safety, including the absence of significant ARIA-E
Finally, ALZN-002 (Alzamend Neuro), which has received regulatory approval to initiate a clinical study, is a cell-based therapy, based on patient-derived dendritic cells pulsed with a mutated Abeta1-42 peptide. This approach has been taken to generate new T-cell epitopes to improve immunogenicity. However, while it seems that the mutations inactivate some of the Abeta T-cell epitopes, it is unclear whether other Abeta T-cell epitopes are still present and thus could be potentially harmful in this therapeutic approach.
Taken together, the accumulating data for vaccines demonstrates general favourable safety, including the absence of significant ARIA-E. Very different approaches are being assessed (eg, liposomes, conjugates, peptide-based, cell‑based) to maximise the level of the induced antibody response, which is particularly important in elderly patients with declining efficiency of the immune system. Some approaches are also focused on targeting specific Abeta conformations (eg, ACI-24.060, ALZ-101). We eagerly await further clinical trial results for the different vaccine candidates to see which of the approaches has the highest potential to succeed in modifying the course of disease.
Active immunotherapies provide a broad range of applications
Independent of the approach used, a question frequently arises regarding how to use active anti‑Abeta immunotherapies, considering that part of the population will already be treated with the approved, or soon-to-be approved, mAb-based therapies. Following mAb treatment discontinuation, Abeta plaques reaccumulate at a slow rate. Modelling for both lecanemab and donanemab suggests that after treatment discontinuation, brain amyloid reaccumulation to baseline levels is slow with a recovery half‑life of approximately four years. However, other biomarkers such as plasma Abeta1-42/1-40 ratio and p-tau181 return to baseline levels faster than amyloid.9 In these cases, it seems logical to envisage vaccination as a maintenance active immunotherapy following mAb therapy. Once the pathological Abeta has been cleared by mAb, antibodies targeting pathological Abeta induced with the active immunotherapy would potentially act by immediately removing newly‑formed pathological components and preventing the establishment of new amyloid plaques.
As mentioned, the clinical benefit of currently available anti-Abeta mAbs is limited, delaying cognitive decline by a few to several months. This could certainly be improved by starting the treatment earlier in the course of disease, before the pathology becomes established. However, this would be difficult to achieve with the costly mAb treatments, where we know that payors typically only accommodate treating an existing pathology as opposed to preventing an imminent pathology.
In this context, active immunotherapy through vaccination could play a significant role. Being more convenient for the patients, less expensive and, therefore, more accessible to the broader population, active immunotherapy could be applied in at-risk populations, before the development of symptoms, or even in a more global population in a fully preventative setting.
Active immunotherapy for other diseases
All these options offer avenues for a more robust programme of treatment and prevention of Alzheimer’s disease. But its power does not end here. The same concept has started to be explored for other neurodegenerative disorders, such as Parkinson’s disease. More globally, where the accumulation of pathological forms of ‘self proteins’ causes disease, the active immunotherapy approach could be considered for different kinds of chronic diseases to modify their course and improve patients’ lives.
About the authors
After obtaining her master’s degree in Molecular Biology and Biotechnology at the Faculty of Biology, University of Belgrade, Serbia, Marija earned her PhD in Neurosciences at University of Lausanne, Switzerland, working on neuronal ion channels. Continuing her work in the neuroscience field, she carried out further postdoctoral research working on neuronal stem cells at the University of Geneva. From there her research shifted to the immunology field, specialising in haematopoietic stem cell transplantation and vaccinology at Geneva University Hospitals. Marija joined AC Immune SA in Lausanne in 2011 as a research scientist and subsequently became a team leader in vaccine discovery. Since 2019, she has been Head of Vaccines and Immunoanalytical Sciences at AC Immune.
After obtaining his PhD in Neuroscience at the Ecole Normale Supérieure de Paris, France, Yves worked on sensory processing at the Ecole Polytechnique Fédérale in Lausanne (EPFL), and dopaminergic networks underlying reward-based learning at the University of Geneva, Switzerland. He then worked as a clinical scientist for a med tech company developing spinal cord stimulation for people living with spinal cord injury. Yves joined AC Immune in 2020 and is now Manager, Investor Relations and Communications.
Marie Kosco-Vilbois joined AC Immune as Chief Scientific Officer in 2019. She has extensive experience in the biopharmaceutical industry and previously served as Chief Scientific Officer for Novimmune from 2005. Prior to joining Novimmune in 2002, she was Head of Immunology and Preclinical Pharmacology at the Serono Pharmaceutical Research Institute, a Senior Scientist and then Head of Immunology at the Glaxo Wellcome Research Institute in Geneva, and a Scientific Member of the Basel Institute for Immunology. Dr Kosco-Vilbois holds a PhD in Anatomy and Immunology from the Medical College of Virginia/Virginia Commonwealth University School of Medicine, US.
In 2003, Andrea Pfeifer co-founded AC Immune SA. She is currently CEO and successfully led the IPO on Nasdaq in 2016. She currently serves as the Chair of Investment Fund BioMedInvest and AB2 Bio SA and is a member of the Supervisory Board of Symrise AG. Andrea is also a key member of the CEOi initiative on Alzheimer’s Disease and the Davos Alzheimer’s Collaborative. In 2021, she was awarded the first SEF.WomenAward for CEO of the Year by the Swiss Economic Forum. Andrea holds a PhD in Toxicology (Cancer Research) from the University of Würzburg, Germany and is a registered Toxicologist and Pharmacist.
References
- Hendrix S. 2023 AD/PD Conference. An overview of clinical trial results in Alzheimer’s Disease in the past 2 decades with a focus on time savings.
- Landry I, Kanekiyo M, Aluri J, et al. 2022 Alzheimer’s Association International Conference. Lecanemab (BAN2401) infusion reactions and immunogenicity: results from randomized phase 2 study and an open-label extension.
- Lowe SL, Duggan Evans C, et al. 2021 Donanemab (LY3002813) Phase 1b Study in Alzheimer’s Disease: Rapid and Sustained Reduction of Brain Amyloid Measured by Florbetapir F18 Imaging. Journal of Prevention of Alzheimer’s Disease. 2021;1–11.
- Vellas B, Black R, Thal L, et al. 2009. Long-Term Follow-Up of Patients Immunized with AN1792: Reduced Functional Decline in Antibody Responders Current Alzheimer Research. 2009;6(2):144–51.
- Gilman S, Koller M, Black RS, et al. Clinical effects of a immunization (AN1792) in patients with AD in an interrupted trial. Neurology. 2005;64(9):1553–62.
- Monsonego A, Zota V, Karni A, et al. Increased T cell reactivity to amyloid β protein in older humans and patients with Alzheimer’s disease. Journal of Clinical Investigation. 2003;112(3):415–22.
- Vukicevic M, Fiorini E, Siegert S, et al. An amyloid beta vaccine that safely drives immunity to a key pathological species in Alzheimer’s disease: pyroglutamate amyloid beta. Brain Communications.2022;4(1).
- Wang CY, et al. UB-311, a novel UBITh® amyloid β peptide vaccine for mild Alzheimer’s disease. Alzheimer’s & Dementia: Translational Research & Clinical Interventions. 2017;3(2):262-272.
- Hayato S et al. Population pharmacokinetic-pharmacodynamic analyses of amyloid positron emission tomography and plasma biomarkers for lecanemab in subjects with early Alzheimer’s disease. CPT Pharmacometrics Syst Pharmacol. 2022;11(12):1578-91.