Maximising the efficiency and application of automated planar patch clamp electrophysiology
Posted: 21 September 2007 | | No comments yet
The widespread expression of ion channels and their ability to significantly modulate cell function makes them attractive drug targets1. Therapeutic agents which target ion channel proteins comprise the third best selling class of prescription drugs with US sales in 2002 estimated at $12 billion. Somewhat surprisingly the discovery of many marketed ion channel modulators was serendipitous rather than rational and these therapies target relatively few (less than 10) of the 400 ion channel genes contained within the human genome2.
The widespread expression of ion channels and their ability to significantly modulate cell function makes them attractive drug targets1. Therapeutic agents which target ion channel proteins comprise the third best selling class of prescription drugs with US sales in 2002 estimated at $12 billion. Somewhat surprisingly the discovery of many marketed ion channel modulators was serendipitous rather than rational and these therapies target relatively few (less than 10) of the 400 ion channel genes contained within the human genome2.
The widespread expression of ion channels and their ability to significantly modulate cell function makes them attractive drug targets1. Therapeutic agents which target ion channel proteins comprise the third best selling class of prescription drugs with US sales in 2002 estimated at $12 billion. Somewhat surprisingly the discovery of many marketed ion channel modulators was serendipitous rather than rational and these therapies target relatively few (less than 10) of the 400 ion channel genes contained within the human genome2.
The progression of ion channels as targets within a drug discovery paradigm has not progressed at the same rate as other target classes such as GPCRs and enzymes as evidenced by new drug registrations. A number of factors are cited to have contributed to the lack of novel ion channel modulators entering clinical utility in the last two decades and arguably these are closely interrelated:
- Ion channels can be difficult and complex to validate as drug targets
- Ion channel pharmacology is commonly poor, exemplified by low potency, non-selective compounds
- The assay technologies historically available to prosecute ion channels have not been ideally suited to the HTS approaches adopted by modern pharmaceutical companies
Patch clamp electrophysiology, with its ability to provide high resolution recordings of small channel currents from a single cell under voltage clamp conditions, remains the gold standard technique. However it is traditionally a time consuming low through-put process, requiring technically skilled operators and has represented a significant bottleneck for many ion channel directed drug discovery projects. Indirect assay readouts such as ion sensitive and potentiometric fluorescent dyes, radiotracer flux and binding assays have significantly higher through-puts but have well documented limitations which can deliver high false positive and negative rates3. Although these latter techniques may be well suited to frontline screening in terms of their through-put and cost effective generation of data points, their fidelity can dictate that electrophysiological follow up is required. Even a respectable hit rate of 0.1% when screening a library of 500,000 compounds, a relatively small screening deck by current standards, would be enough to occupy most company’s electrophysiology capabilities for an uneconomic period of time. This bottleneck, along with the promise of employing electrophysiology in frontline hit finding and as primary support for lead optimisation efforts, has been one of the major drivers for the development of automated electrophysiology systems. These systems, in the majority attributable to the advent of planar patch clamp technique4, are beginning to have a major impact in the field – however they do raise challenges of their own in maximising their cost effectiveness and efficiency, particularly with respect to the cell-based reagents they require for success.
Conventional versus planar patch clamp electrophysiology
One of the key advantages of the patch clamp technique is widespread applicability to almost every cell type. Several other electrophysiological techniques require the impalement of the cell under study by one or more sharp glass electrodes (for example, twin-electrode voltage clamp) with minimal damage to the plasma membrane. This limits their application to relatively large cells with a significant cross sectional profile hence cells such as red blood cells (small size) and endothelial cells (flat and thin) are not amenable to such approaches. With patch clamping this limitation is overcome as the patch pipette, which in electrophysiological terms is relatively blunt (1-2um tip diameter compared to 0.1um for a ‘sharp’ electrode used for impalement), is gently placed upon the cell surface to facilitate recording rather than impaling it.
An additional component of conventional patch clamp success rates is the refining and optimisation of the general technique (for example, facilitating the formation of the high resistance giga-ohm seal between the patch pipette and cell surface) which is commonly required for individual cell types. Both successful recording rate and seal resistance magnitude can be strongly dependent upon a combination of parameters that the patch clamper can dynamically adjust. These include; patch pipette shape, pipette resistance, application of pressure to the pipette during approach to the cell, where to place the pipette on the cell membrane and the level of negative pressure to apply during seal formation, to name but a few. Even with cell lines commonly used for heterologous channel expression many patch clampers will subjectively select the cells for study based upon their morphological appearance when viewed under the microscope citing the fact the cells look ‘healthy’ (clean, unruffled membranes, non-vacuolated cytoplasm) or that experience has taught that a certain morphology correlates with high channel expression (for example, ‘neurite’ like extensions, pseudopodal outgrowths).
With the planar patch clamp technique the patch pipette has been replaced by an etched or drilled aperture in a flat substrate which forms an integral component of the well or plate/chip (Figure 1). As with patch pipettes these are commonly single use and disposable, providing the majority of the cost of running an automated planar patch clamp system (cost varying from $0.5 – $10 per data point depending upon the system). In contrast to the conventional technique where the patch pipette is moved to the cell to be studied (usually adhered to a glass coverslip) planar patch clamp systems position the cell (which is in suspension) to the aperture using suction and/or electrical positioning. To date manufacturers supply plates with only a single type of aperture geometry and this cannot be adjusted or optimised by the end user. Additionally the mostly commonly utilised planar systems, the IonWorks HT/Quattro5 (Molecular Devices), Patch Xpress (Molecular Devices)6 and the Qpatch 16/HT7 (Sophion Biosciences), both add several thousand cells to each well to facilitate sealing rates – the cell that is eventually sealed and studied is ‘blindly’ selected at random. As such the approach is commonly to optimise the cells to the system rather than vice-versa.
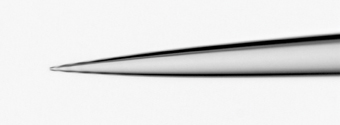
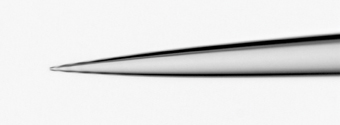
Figure 1a: Comparison of glass patch pipette with planar patch substrate. A. Conventional glass patch pipette pulled from borosilicate glass – tip opening is 1µm diameter
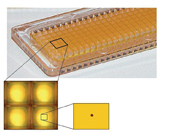
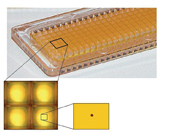
Figure 1b: Planar patch plate as utilized by the IonWorks platform – this is a 384 well substrate, based on a 1536 SBS plate well geometry. The standard single cell patch plates contain a single aperture in each well forming the recording site.
Defining success with automated electrophysiology platforms
With planar patch systems, success rates are commonly defined at key steps of the recording process, such as, formation of an appropriate seal between cell and planar substrate (for example, >50MOhm for the IonWorks platform, ~1 Gohm for the PatchXpress and Qpatch systems) which is maintained through-out the recording and a whole cell current attributable to the channel of interest at an appropriate magnitude. The relatively low resistance seals formed on the IonWorks platform commonly dictate that higher levels of channel expression are required for good resolution (greater than several hundred pA) compared to more conventional higher resistance systems which can accurately resolve lower currents (20-30pA in some cases).
Optimisation of seal rates
A range of cell backgrounds have been successfully employed for use on automated planar patch systems including CHO, HEK, CHL and RBL cells, common backgrounds for heterologous ion channel expression. In many cases CHO lines demonstrate superior performance widely believed to be due to their ‘clean’ membrane and low potential for forming multicellular ‘clumps’ once detached from their culture substrate.
Cell husbandry is a key determinant of platform performance – harvesting cells during their logarithmic growth phase using an optimised/defined procedure is common. With conventional patch clamp the cells are usually seeded onto glass coverslips and kept in culture for 4 – 72h prior to use, thus the method of detachment from culture flasks commonly has little influence on seal rates. Planar patch systems use the cells within minutes (IonWorks) to hours (Qpatch) of their detachment and thus the method and reagent utilised can strongly influence outcome. Trypsin may be optimal for some lines but a variety of non-enzymatic protocols using reagents such as Versene (Invitrogen, UK) and Detachin (Genlantis, USA) or a combination of each with trypsin in differing proportions have been reported by vendors and users alike as a key to enhanced recording success. The end goal is to produce an even homogenous suspension of cells with no or few clumps – trituration to resuspend cells both on and off the instruments can affect seal rates profoundly. The authors experience with the IonWorks platform suggest that a ‘bell shaped curve’ exists with many cell lines which require some trituration but an excessive amount leads to a loss of performance with respect to seal rates. If the cell line to be studied does exhibit clumps then removing these by sieving with a fine mesh filter (for example, 70µm cell strainers; Becton Dickinson, USA) can abrogate potential problems.
The density of the cells seeded into the planar patch plate is an additional consideration. Most systems use on board fluidics to pipette µL volumes of cell suspension into each recording site (well or chip) commonly in the range of 1,000 to 10,000 cells per recording site. Despite only a single cell being eventually sealed, this high number of cells is required to maximise the probability of a cell being positioned over the recording aperture. It is this characteristic that underlies the significant appetite most automated planar patch systems exhibit for cells, requiring several million cells per experimental run – a stark contrast to a conventional patch clamp rig which requires very few cells (several hundred seeded onto glass coverslips). In many cases, an increase in cell density correlates to an increase in seal rates although this is not a linear relationship with very low and very high densities impacting negatively on performance. This parameter is a particularly important consideration with the IonWorks HT/Quattro platform which is an ‘add and read’ system. The platforms inability to superfuse the recording sites once seals are formed means that all of the cells placed in the recording well remain present for the duration of the experimental protocol. A high number of cells, commonly coupled to a high level of channel expression per cell, can lead to a reduction in the assumed free concentration of any added compound(s) to the well – a phenomena termed ligand or compound depletion. This can result in aberrant pharmacology in comparison to conventional patch clamp, evidenced by right-shifted concentration-response curves. For this platform an optimal cell density is usually experimentally determined to balance seal rates with pharmacological fidelity. Other platforms such as the Qpatch overcome this limitation by perfusing away excess cells from the recording site prior to any compound additions.
Choice of recording solutions can influence seal rates. In common with conventional patch clamp, divalent cations appear to be a pre-requisite for seal formation using planar patch substrates – increasing the concentration of Ca2+ or Mg2+ in the external solution can provide higher resistance seals, a potential benefit with the caveat that this manouevre does not impact upon the channel under study. Others have reported that the partial substitution of NaCl with NaF has produced significant increases in seal resistances and recording success rates.
Even when cell husbandry protocols and recording solutions are optimised, not all cell lines or even clones will perform identically. The high through-put nature of automated planar systems allows many of the parameters discussed above to be assessed in parallel with significant experimental determinations for each condition which could not have previously been achieved with conventional patch clamp techniques. The IonWorks platform uniquely allows seeding of multiple sections of a single 384 well recording plate with up to 24 different cellular preparations or cell lines/clones by the use of multiwell ‘cell boat’. Figure 2 shows data from a single CHO cell line stably expressing a potassium channel subsequent to single cell sorting and clonal expansion – functional screening of 48 clones under identical conditions shows the significant variance in seal success rates despite no overt differences in cell morphology or growth characteristics.
Figure 2: Functional screening of ion channel clones by planar patch clamp electrophysiology. Data are shown for 48 cell clones isolated from a stable cell line expressing a Ca2+-dependent potassium channel (CHO-BKCa). Single cells were expanded initially in 96 well plates and subsequently 25cm2 culture flasks prior to testing on the IonWorks platform. Upper panel shows peak current amplitudes and lower panel seal rates (defined as percentage exhibiting seals > 50MWΩ). Data are expressed as mean ± s.e.m., n =32 per clone.
Channel expression rate and seal rate can conspire to undermine success
The ideal cell line for use with automated planar platforms would be one in which every cell expressed the channel of interest at an identical and appropriate level. Unfortunately channel cell lines rarely approach the ideal situation and even clonal lines can demonstrate significant heterogeneity with respect to their expression profile (see Figure 2b & Figure 3b). Cells which successfully seal but have low or no current expression can significantly undermine overall instrument performance. Additionally heterogeneous expression can undermine application of these platforms to assays designed to measure positive modulators8. The combined contribution of both suboptimal seal and expression rates led to Molecular Devices recommending that compounds were screened in quadruplicate on their first generation IonWorks HT platform to guarantee at least a single successful recording was obtained for each compound5. This limitation still hinders the majority of the planar systems and can result in overall recording rates for experiments to drop as low as 50% of the recording sites seeded with cells. Consequentially reduced through-put and incremental increases in cost per effective datapoint are the end result. The second generation IonWorks platform, the Quattro, has employed a unique approach which delivers dramatically enhanced success rates – Population Patch Clamp (PPC)9. PPC permits the recording from multiple cells per recording site by employing planar plates which have 64 apertures per well (see Figure 1). The measured current is the sum of currents from each individual cell and is subsequently divided by 64 to provide an ‘averaged’ single cell current. The benefits of PPC recording over single cell automated electrophysiology are two fold:
- Provided a high percentage of the 64 recording apertures are occupied by cells with high resistance seals, a number of open or apertures with low resistance seals can be tolerated. The result is an enhanced number of wells with a mean seal resistance acceptable for recording.
- Cell lines with heterogeneous channel expression levels can be tolerated as long as the measured mean current is adequate. Low or none-expressing cells, if in the minority, will be offset by those with significant expression. Additionally well to well variability will be significantly reduced (Figure 3b).
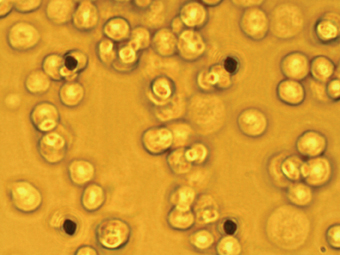
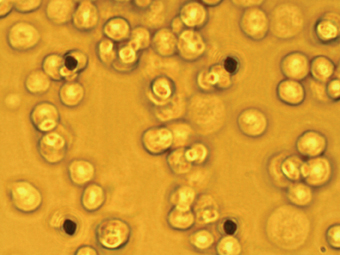
Figure 3a: Population patch clamp (PPC) recording. A IonWorks Quattro PPC planar patch plate – 64 apertures are clearly visible as an 8 x 8 array.
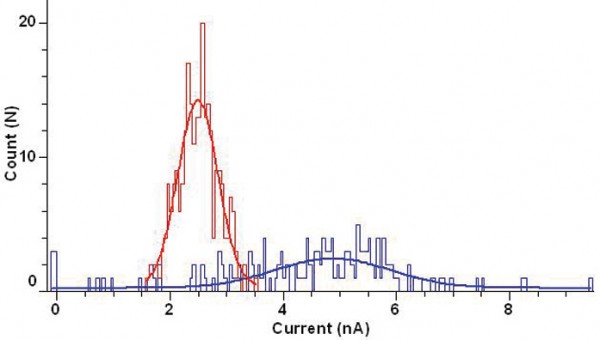
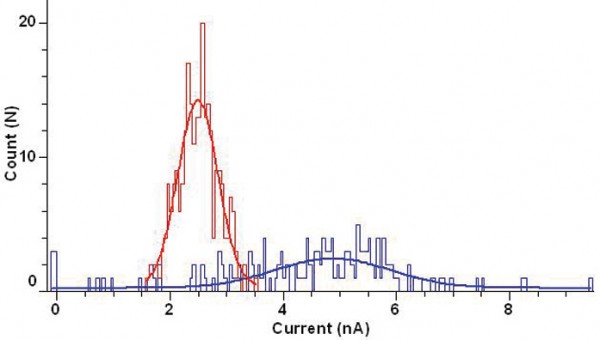
Figure 3b: Cell population distribution histograms of peak current amplitudes for stable CHO-BKCa cell line (bin width 0.1nA) recorded using the IonWorks Quattro. Blue lines show distribution recorded using standard single cell (HT) substrate and red lines the distribution recorded using PPC – note the tighter distribution with PPC and lack of wells exhibiting current <1nA. Data are fitted with a single Gaussian fit.
These two characteristics of the PPC technique combine to offer success rates for recording which can approach 100%, thus screening of one compound per recording well is a feasible reality. Additionally the significant reduction in variability in well to well current amplitude is proving empowering for assays designed to screen for positive modulators (channel openers or activators)8. Preserving success The need for high cell numbers per experiment required by most planar patch clamp systems (106 – 107 cells for the IonWorks platform) can place a considerable burden upon cell culture, particularly if the instrument is being utilised at full or near full capacity (~8-10 experimental cycles per day). This requires preparation of relatively large cell batches which despite the best efforts of a rigorous culture routine, can display passage to passage variation in sealing rates and current magnitude which can undermine platform performance. Although many vendors recommend that cells are prepared ‘freshly’ immediately prior to use, the authors approach to minimise inter-experimental variability and maximise cell utility has been to cryopreserve cells. Batches of cells functionally confirmed on the automated platform, are frozen in aliquots in growth medium supplemented with DMSO and maintained at -80°C. When required cells are rapidly thawed, washed to remove the DMSO and used immediately, the entire cell preparation process takes a matter of minutes. The authors experiences with a number of CHO and BHK cell lines show that seal rates, current magnitudes and pharmacological responses show no significant differences from freshly prepared cells even when used 2 months after freezing (see Figure 4). This approach has a number of advantages over fresh cell preparation: • Cell performance is almost completely predictable • Cell wastage is minimal – frozen aliquots contain the exact number of cells required for a single experimental run • Preparation time is reduced – fresh preparation requires detachment from substrate, centrifugation, re-suspension and counting prior to readiness for use • Preparing large batches offers economies of scale with respect to tissue culture in terms of both reagents and resource.
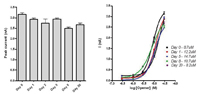
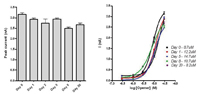
Figure 4: Utility of cryopreserved cells for automated electrophysiology. CHO-BKCa cells were frozen as aliquots in growth media supplemented with DMSO and maintained at -80°C for up to 20 days. On the indicated days cells were rapidly thawed at 37°C, washed and re-suspended in extracellular buffer immediately prior to recording on the IonWorks platform. Cell line performance was unaffected by cryopreservation for up to 20 days as indicated by no significant changes in either peak current amplitudes (A) or pharmacological response to a known channel activator (B). Data are mean ± sem (A, n > 64 for each time point; B, n = 8 for each opener concentration).
Transient expression – an alternate approach?
Not all ion channels are readily amenable to stable expression – multiple subunit channels can be particularly challenging to generate appropriate stable lines, whilst some channels induce toxicity if expressed at levels required to give good signal to noise ratios in electrophysiological experiments (for example, some K channels, ENaC). One approach to overcome this limitation with conventional patch clamp electrophysiology is to transiently express the channel of interest. Utilising transfection regeants such as lipofectamine or FuGENE, which have a relatively low efficiency rate (30-50% of cells) can require co-expression of a fluorescent marker such as green fluorescent protein (GFP), which allows the patch clamper to positively select channel expressing cells to study. These reagents can be used to prepare transiently expressed cells for study by planar patch clamp, but the low efficiency of expression and the inability of the platforms to select the cell for study requires that cells must be sorted (by fluorescence activated cell sorting; FACS) prior to use to gain acceptable numbers of recordings from cells with current (Figure 5). This approach is not well suited to routine experimental practice as sorting can be both labour and time intensive even with a high speed FACS system. When combined with low transfection efficiency, which means that as much as 70% of cells cultured will not be suitable for usage, this places an enormous burden upon cell preparation.
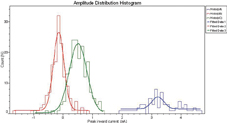
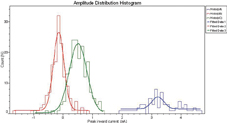
Figure 5: Using transiently transfected cells for planar patch clamp electrophysiology. Wild-type CHO cells were transiently co-transfected with the α,β and γ subunits of the epithelial sodium channel (ENaC) and eGFP, and functionally screened 24h later for the presence of ENaC using the IonWorks Quattro and Population Patch Clamp. Cells were screened immediately after detachment from the culture flask (non-sorted) or subsequent to sorting for eGFP expression by high-speed fluorescence-activated cell sorting (FACS). Note that current amplitude and hence channel expression correlates with eGFP expression. Non-sorted cells show a mean peak current of ~0.5nA but the high number of wells with currents <250pA make these non-viable for routine use
Virally mediated transfection systems offer much improved efficiencies. Baculovirus mediated transfer of cDNAs into mammalian cells achieved via insertion of a human promoter into the insect virus (‘BacMam’ virus), is a relatively recent development10. This has been successfully utilised to transiently express the ATP-sensitive potassium channel (KATP), a multimeric complex of the sulphonylurea receptor (SUR) and an inward rectifier K channel subunit (Kir 6.2)11. Preliminary studies undertaken by the authors using the BacMam approach to transiently express ENaC, a multimeric complex of 3 subunits (α‚ β and γ), have proven successful in generating measurable currents on the IonWorks platform.
Summary and outlook
Automated planar patch clamp systems are already making a significant impact as an enabling technology in the ion channel drug discovery arena. The technology is facilitating the generation of high quality electrophysiological data at rates orders of magnitude higher than traditional pipette-based approaches have offered. The systems do require robust and, on occasion, bespoke cell-based reagents for maximum efficiency but do, by virtue of their through-put, offer the ability to functionally screen numerous lines and clones to identify the most appropriate. The knock on effect of this is the development of better reagents for use in conventional electrophysiology. Several vendors are now utilising these systems to develop cell lines for commercial purpose and in some cases these lines are provided already optimised for use by planar patch systems.
Many in the field expect that the next generation of planar patch systems will have further increased through-put (to date the 384 well IonWorks Quattro is the highest) with the aim of utilisation in frontline HTS campaigns employing considerable screening decks. The successful implementation and utility of the current technologies is creating a new branch of patch clamp electrophysiology, almost a discipline of its own. This technology is gaining wider adoption both within the pharmaceutical and biotech industries and looks to have a bright future.
Acknowledgements
The authors would like to thank Anne Dodge and Laura Clarke for their contributions in establishing the CHO-BKCa IonWorks assay.
References
- Ashcroft FM (2006) From molecule to malady. Nature 440(7083); 440 – 447.
- Li S, Gosling M, Poll CT, Westwick J & Cox B (2005) Therapeutic scope of modulation of non-voltage gated cation channels. Drug Discovery Today 10(2); 129 – 137.
- Treherne JM (2006) Exploiting high throughput ion channel screening technologies in integrated drug discovery. Current Pharmaceutical Design 12; 397 – 406.
- Fertig N, Blick RH & Behrens JC (2002) Whole cell patch clamp recording performed on a planar glass chip. Biophysics Journal 82(6); 3056 – 62.
- Schroeder K, Nagel B, Trsize DJ & Worley J (2003) IonWorks HT: a new high-throughput electrophysiology platform. Journal of Biomolecular Screening 8(1); 50 – 64.
- Dubin AE, Nasse N, Rohrbacher J, Hermans AN, Marranes R, Grantham C, Van Rossem K, Cik M, Chaplan SR, Gallacher D, Xu J, Guia R, Byrne NG & Mathes C (2005) Identifying modulators of the hERG channel activity using the Patch Xpress planar patch clamp. Journal of Biomolecular Screening 10(2); 168 – 81.
- Mathes C (2006) Qpatch: the past, present and future of automated patch clamp. Expert Opinions in Therapeutic Targets 10(2); 319 – 27.
- John VH, Dale TJ, Hollands EC, Chen MX, Partridge L, Downie DL, Meadows HJ & Tresize DJ (2007) Novel 384-well population patch clamp electrophysiology assays for Ca2+-activated K+ channels. Journal of Biomolecular Screening 12(1); 50 – 60.
- Finkel A, Wittel A, Yang N, Handran S, Hughes J & Costantin J (2006) Population patch clamp improves data consistency and success rates in the measurements of ionic currents. Journal of Biomolecualr Screening 1(5); 488 – 96.
- Kost TA, Condreay JP, Ames RS, Rees S & Romanos MA (2007) Implementation of BacMam virus gene delivery technology in a drug discovery setting. Drug Discovery Today 12(9-10); 396 – 403.
- Pfolh JL, Worley JF, Condreay JP, An G, Apolito CJ, Kost TA & Truax JF (2002) Titration of KATP channel expression in mammalian cells utilizing recombinant baculovirus transduction. Receptors Channel 8(2); 99 – 111.
Martin Gosling
Martin Gosling completed his PhD at the University of Aston in Birmingham studying chloride channels in osteoblasts in 1995. After 3 years as a post-doctoral research fellow studying ion channels in vascular endothelium at Charing Cross & Westminster Medical School, he obtained a tenured position as Lecturer in Vascular Physiology at Imperial College School of Medicine and continued to study the role of ion channels in mechanotransduction. In 2001 he joined Novartis in the Respiratory Diseases Area and over the last 5 years has established and led the Ion Channel Pharmacology Group.
Paul Groot-Kormelink
Paul Groot-Kormelink studied Biochemistry at the University of Nijmegen, The Netherlands. He obtained his PhD at the Faculty of Medicine, University of Leuven (Belgium) studying the assembly and stoichiometry of neuronal nicotinic acetylcholine receptors. During his post-doctoral years (1998 to 2005) at University College London he continued working on ligand-gated ion channels in the groups of Dr Lucia Sivilotti and Prof David Colquhoun. He joined Novartis in 2005 and in his current role is responsible for the tool provision of the Ion Channel Pharmacology group.
Pamela Tranter
Pamela Tranter graduated with a BSc in Biotechnology from Queen Elizabeth College, London. She studied her PhD at the Royal Free Hospital School of Medicine on the signalling pathways involved in platelet activation by LDL. She joined the Thrombosis Unit at Ciba-Geigy in 1989 and subsequently transferred to Respiratory Disease when the company merged to form Novartis. She is currently responsible for the automated electrophysiology support within the Ion Channel Pharmacology Group.