Calorimetry for polymorph detection
Posted: 27 March 2007 | | No comments yet
Characterising the properties of a material, understanding how these properties change in relation to local environment and quantifying potential interactions with other species are facets central to any drug development programme. Not understanding and, more importantly, not controlling these factors can have serious consequences for a pharmaceutical, from irreproducible processing to poor bioavailability, product instability and, worse, patentability. Properties that may be characterised include solubility, dissolution rate, stability (in combination with other excipients and as a function of relative humidity and temperature) and per cent crystallinity (or amorphous content).
Characterising the properties of a material, understanding how these properties change in relation to local environment and quantifying potential interactions with other species are facets central to any drug development programme. Not understanding and, more importantly, not controlling these factors can have serious consequences for a pharmaceutical, from irreproducible processing to poor bioavailability, product instability and, worse, patentability. Properties that may be characterised include solubility, dissolution rate, stability (in combination with other excipients and as a function of relative humidity and temperature) and per cent crystallinity (or amorphous content).
Characterising the properties of a material, understanding how these properties change in relation to local environment and quantifying potential interactions with other species are facets central to any drug development programme. Not understanding and, more importantly, not controlling these factors can have serious consequences for a pharmaceutical, from irreproducible processing to poor bioavailability, product instability and, worse, patentability. Properties that may be characterised include solubility, dissolution rate, stability (in combination with other excipients and as a function of relative humidity and temperature) and per cent crystallinity (or amorphous content).
New analytical tools are constantly being developed and there is a wide array of techniques with which to interrogate pharmaceutical substances. Popular among these are those classified under ‘Thermal Analysis’; of these, calorimetric techniques, particularly differential scanning calorimetry (DSC), are very widely used because of the unique set of qualities they offer. It is the purpose of this review to look at the relationships of calorimetric techniques to other thermal analysis apparatus; to explain the basic operating principles of the apparatus; to explore the roles, advantages, disadvantages and limitations of calorimetry in the pharmaceutical arena and to update the reader with the very latest developments in this important and exciting field. This article focuses on applications to polymorphism; in the next issue the focus will be on quantification of small amorphous contents (May 2007 issue).
Heat as an indicator of change
Any review should start with some definitions; here, thermal analysis is taken to mean any experimental measurement made on a sample while that sample is subject to a defined temperature programme.1 Techniques such as thermogravimetry (TG), dynamic mechanical analysis (DMA), dielectric spectroscopy and thermally stimulated current (TSC) fall under this definition; they are related because they impose some temperature programme upon their samples but they all measure different properties. When the measured property is a change in heat21 then the technique is calorimetric (calor, L – heat; -metry, Gr – measurement); there are a number of popular instrumental arrangements (DSC, noted above, isothermal calorimetry and solution calorimetry) and calorimetric techniques can be considered as a subset within thermal analysis.
Calorimetry thus means simply the measurement of heat (more correctly the measurement of a change in heat content); so why are calorimetric techniques so useful? Simply put, heat is a universal accompaniment to chemical or physical change. In other words, it is possible to study virtually any event by calorimetry because it will invariably occur with a change in heat content. Moreover, the calorimeter operates invariantly to sample physical form, which means that even complex formulated products can be investigated. The decision as to whether calorimetry is a suitable tool to investigate a sample then reduces to the following factors:
- Sensitivity
- Sample size
- Data complexity
Dealing with sensitivity first, it is impossible to define the sensitivity of a calorimetric measurement for a particular sample ahead of time unless the enthalpy change (ΔH) for the measured process is known. The larger the ΔH value the more sensitive the instrument. Sample size is often a crucial consideration for pharmaceuticals where only a few milligrams of a material may exist. Most DSC instruments utilise sample masses of ca. 2-5 mg. Other instruments, such as isothermal or solution calorimeters, require slightly larger masses, ca. 50 – 100 mg. Of course, these may be reduced if the ΔH value is high (which is often the case for crystallisation, for instance). As noted above, the ability to study complex, heterogeneous samples is a strong asset and is no problem if the whole sample fits within the calorimetric ampoule. If this is not the case, then a representative fraction of the sample can be loaded instead.
The issue of data complexity is more fundamental and can be considered as one of the factors that has limited the more widespread application of calorimetric apparatus. It is evident that spectroscopic data are resolved into intensities at specific wavelengths; thus, it is possible to identify and quantify specific species in mixed samples. Heat, conversely, does not come in different colours and, hence, one measures the net change in heat content from all the processes that have occurred in the sample ampoule. The challenge lies in deconvoluting the data into their component parts and in ensuring that careless experimental design or analysis does not result in misinterpretation of the events undergone by the sample. Gaisford and O’Neill have discussed recent advances in these analyses and the reader is referred there for further discussion.2
Types of calorimetry
Having established why calorimetric techniques are so versatile, it is appropriate to explain briefly the operating principles of the most common forms, since this aids the subsequent comparison of data and discussion. Calorimeters can be classified as temperature scanning or isothermal but share common measurement principles.
Measuring principles
There are only three methods by which heat can be experimentally measured:
- Measurement of the power required to maintain isothermal conditions in a calorimeter, the power being supplied by an electronic temperature controller in direct contact with the calorimeter (power compensation calorimetry)
- Measurement of a temperature change in a system which is then multiplied by an experimentally determined cell constant (adiabatic calorimetry)
- Measurement of a temperature difference across a path of fixed thermal conductivity which is then multiplied by an experimentally determined cell constant (heat conduction calorimetry)
Note that all calorimetric measurements therefore require a minimum of two experiments (one for measurement and one for calibration) although further measurements may be needed for blank corrections (such as the determination of a baseline or the correction for dilution enthalpies in a titration experiment).
Temperature scanning calorimetry
The most commonly encountered form of calorimetry, certainly in pharmaceuticals, is differential scanning calorimetry (DSC). In DSC the power output from a sample is measured, relative to an inert reference, as it is heated or cooled in accordance with an underlying temperature programme. The temperature programme can be linear, modulated by some mathematical function (temperature-modulated DSC, TM-DSC,3) operated at fast scan rates (fast-scan DSC, FS-DSC) or modified by the instantaneous sample reaction rate (sample-controlled thermal analysis, SCTA.4 ). DSC data can be presented in a number of ways; most commonly power is plotted versus temperature, although it is also common to plot heat capacity versus temperature (obtained by dividing the power data by the heating rate). Noble provides an excellent discussion of the relative merits of DSC.5
It is also possible to increase the sample temperature in defined steps, so termed ‘step-isothermal’. It is possible to operate some DSC instruments in step-isothermal mode as well as some isothermal instruments.
Isothermal calorimetry
In isothermal calorimetry (IC), data are recorded as the sample is held at a constant temperature. In the simplest case sample and reference materials are placed in sealed ampoules. Ampoules can be reusable or disposable, can vary in volume and can be constructed from a variety of materials (typically they are either glass or metal). In batch calorimetry the reaction vessel is divided into two compartments, which are connected by an air-space. Each compartment can be separately charged with sample (either a solid or a liquid). Once thermal equilibrium has been attained, the contents of the two compartments are mixed, usually by rotating the vessel, and the heat of interaction is measured. Batch calorimeters are also known as mixing calorimeters.
The versatility of isothermal calorimeters derives from the myriad of ways of loading the sample in the ampoule and controlling the local environment. In particular the relative humidity (RH) in the ampoule can be controlled (either by the use of salt solutions in hydrating reservoirs or by perfusing a carrier gas through the ampoule).
Two further derivative forms of isothermal calorimetry are popular; isothermal titration calorimetry (ITC) and solution calorimetry (SC). In ITC small aliquots of a titrant solution (held in a reservoir external to the instrument) are added in sequential aliquots to a solution held within the calorimetric vessel and the heat change per injection is recorded. In SC the heat change when a solute (usually a solid but liquids may also be used) is dispersed in a large volume of solvent (to ensure complete dissolution) is measured. Usually this is achieved using ‘ampoule breaking’ instrumentation (wherein the solute is held in an ampoule which is mechanically broken into the solvent reservoir). Two types of solution calorimeter design are commercially available: instruments that operate on a semi-adiabatic principle (i.e. that record a temperature change upon reaction) and instruments that operate on a heat-conduction principle (i.e. that record a power change directly upon reaction).
Applications in materials characterisation
Polymorph detection
For new APIs, the pressure to bring a product to market usually means that the developing company doesn’t have the time to characterise the relative bioavailability of each polymorphic form; usually, the most stable form is selected for development and the formulation is tailored to ensure its stability. This formulation strategy ensures compliance with the guidance provided by the International Commission on Harmonisation (ICH) on the selection of solid forms of drugs.6 Similarly, for Abbreviated New Drug Applications (ANDAs) the sponsor must provide evidence that the proposed generic product and the original product are pharmaceutically equivalent and bioequivalent;7 Clearly, not having any control or knowledge of the polymorphic forms present would render submission of such data impossible. Calorimetric techniques cannot be used to screen for polymorphs (i.e. an experiment cannot be constructed that will result in the formation of all possible polymorphs; that is usually done by crystallisation from a range of solvents) but can be used to identify and quantify the polymorphic form of a given sample.
Most commonly DSC will be employed for polymorph identification; the method works on the principle that different polymorphs will have different melting temperatures. If the sample is a metastable polymorph, the data will show the melt and re-crystallisation peaks for each metastable polymorph until the stable polymorph is attained. The method is robust, reliable and applicable to virtually any sample, which explains its widespread application8-13.
A good example is that of Carbamazepine, a drug used in the treatment of epilepsy. It has been shown successfully, using DSC, that three distinct polymorphs of Carbamazepine exist.8 These findings were complemented by results obtained from infra-red spectroscopy and powder X-ray diffraction experiments.
However, although DSC has proved its worth in identifying the presence of multiple polymorphs it is not always possible thermally to characterise the lower melting polymorphs of a compound. At the heating rates employed in a typical DSC experiment (ca. 5-20°C min-1) there are often multiple thermal events that are associated with concurrent re-crystallisation to a new form and subsequent melting of that new form. If these thermal events overlap it is almost impossible to separate the data into their component heats. In the case of carbamazepine, in the initial study quantification of the enthalpy of fusion of Form III was not possible because of a concurrent recrystallisation of Form III to the higher melting Form I under slow heating rates.8
Recent advances in technology have permitted the construction of fast scan DSC (FS-DSC) instruments capable of scan rates of up to 500°C min-1. With FS-DSC the sample is heated so rapidly that the data effectively represent a ‘snapshot’ of its form at the start of the experiment (in other words, if a sample contains different polymorphs then during a conventional DSC experiment there is time for the different forms to interconvert but in FS-DSC the melting temperature of a higher melting form is reached before the sample has time to convert to a different form). FS-DSC has been used to study Carbamazepine and it has been shown that as the scan rate increases, recrystallisation to Form I is inhibited, until it is completely retarded at 500°C min-1.14 With the inhibition of the re-crystallisation of Form I it was possible to measure directly the enthalpy of fusion from the calorimetric data, 109.5 J g-1.
McGregor et al14 also describe a method by which application of fast scan rates permits the determination of polymorphic purity. Here binary mixtures of carbamazepine Form III and Form I were prepared in various ratios between 95:5 – 1:99 w/w Form III : Form I in order to determine the limit of detection of Form III in a mixture. They were able to detect levels of 1% w/w Form III present in a mixture with Form I. They note, however, that crystal seeding of Form III by Form I in the mixture results in a partial re-crystallisation of Form III preventing accurate quantitative determination of the quantity of low levels of Form III.
Although it is not possible to quantify accurately the polymorphic purity of a system the ability to discriminate for the presence of 1% w/w polymorphic content is extremely desirable. Many techniques have a limit of detection of approximately 5% w/w, which is often outside the desired detection limit for a pharmaceutical compound. The rapidity of the measurement also offers the significant advantage of time and cost particularly as it allows a rapid screen of a product without the requirement for lengthy and expensive analysis to determine its polymorphic composition.
An alternative to DSC is solution calorimetry. Here, as noted earlier, the heat change upon dissolution of a solid sample is recorded. Solution calorimetry has been used to identify and quantify polymorphs of many drugs. For instance, Forms I and II of cyclopenthiazide have been shown to have comparable enthalpies of solution (~6 kJ mol-1) while Form III has a higher enthalpy of solution of 15 kJ mol-115. Similarly, solution calorimetry has been used to characterise the three polymorphic Forms of a pre-clinical drug, Abbott-7917516 and the polymorphs of an angiotensin II antagonist agent (MK996).17
A study of urapidil resulted in the enthalpies of solution of Forms I and II of the drug (21.96 and 23.89 kJ mol-1 respectively) being determined18. However, the authors note that urapidil exists in an additional Form (Form III), which they were unable to isolate. By measuring the heat of solution of the mixture (ΔmixH) and using DSC data to determine the percentage of each polymorph in a sample containing Form III (7.4% Form I, 2.7% Form II and 89.9% Form III), they calculated the enthalpy of solution of Form III using the following relationship:


Equation 1
where XI, XII and XIII are the fractions of Form I, II and III respectively and ΔHI, ΔHII and ΔHIII are the heats of solution of Forms I, II and III respectively. It was determined that ΔHIII equalled 22.98 kJ mol-1.
It is not usually possible to use isothermal calorimetry to identify the polymorphic composition of a sample, but if the rate of conversion between polymorphs is reasonably fast (the extent of conversion, α, is >0.15 over the lifetime of the experiment) then there is the possibility to study polymorphic behaviour.
Seratrodast (an anti-asthmatic drug) has been studied via an isothermal technique.19 The drug was allowed to convert between polymorphs over a period of approximately 20h, under controlled RHs. Elevated temperatures (50 – 65°C) were used to ensure that a sufficient fraction of the material had converted in order to perform the analysis. It was possible to quantify the enthalpy of transition (-5.70 ± 1.13 x 10-1 kJ mol-1) and understand the kinetic nature of the event by application of the Hancock-Sharp equation:20


Equation 2
Where B is a constant. The Hancock – Sharpe method20 consists of plotting ln[ln(1-α)] versus ln t to obtain a series of straight lines, the slopes of which yield a parameter m. The value of this parameter then allows the prediction of a kinetic model that describes the mechanism of polymorphic transformation. From the values derived (m = 2.00, 2.09 and 2.1) the authors concluded that for each of the three sieved fractions the mechanism of transformation of seratrodast is best described by a two dimensional growth of nuclei model. Using the Avrami-Erofeev equation, below, they also showed that it was possible to calculate the rate constant for the transformation for each sieve fraction and that the rate constant increased as the particle size decreased.
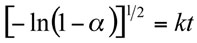
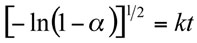
Equation 3
Where k is a rate constant. As the study was conducted as a function of temperature it was also possible to calculate an activation enthalpy, thus permitting the prediction of a shelf-life (based upon 90% of Form II remaining, t90) for a variety of conditions and storage temperatures. For example, it was predicted that t90 for the transition of Form II to Form III was approximately 3900 days at 63% RH and 298K and 17.5 days at 63% RH and 313K.
Summary
Calorimetric techniques offer a number of unique benefits for analysis of pharmaceuticals: measurement of a ubiquitous property and invariance to sample physical form or heterogeneity. They are thus ideally suited to many areas of pharmaceutical development. However, data interpretation is not necessarily straightforward and can be dependent upon sample preparation as well as the type of instrument used. This review provides an overview of the use of calorimetry for polymorph characterisation. It also discusses some of the drawbacks of the techniques and it is hoped that the reader will better appreciate the subtleties of interpretation that are required when making proper use of calorimetric data. In the article to appear in the May Issue, the application of calorimetric methods to quantification of small amorphous contents will be discussed.
References
- Stahl JW. Nomenclature of thermometric and enthalpic methods in chemical analysis (IUPAC recommendations 1994). Pure App Chem 66:2487-2492, 1994.
- Gaisford S, O’Neill MAA. Pharmaceutical isothermal calorimetry. Informa Healthcare (New York) ISBN 0-8493-3155-2, 2006.
- Reading M, Luget A, Wilson R. Modulated differential scanning calorimetry. Thermochimica Acta 238:295-307, 1994.
- Charsley EL, Warrington SB. Thermal analysis: Techniques and applications. Royal Society of Chemistry (Cambridge) ISBN 0-8518-6375-2,1992.
- Noble D. DSC balances out. Anal Chem 67:323A-327A, 1995.
- Grant DJW, Byrn SR. A timely re-examination of drug polymorphism in pharmaceutical development and regulation. Adv Drug Del Rev. 56:237-239, 2004.
- Raw AS, Furness MS, Gill DS, Adams RC, Holcombe Jr FO, Yu LX. Regulatory considerations of pharmaceutical solid polymorphism in abbreviated new drug applications (ANDAs). Adv Drug Del Rev, 56:397-414, 2004.
- Rustichelli C, Gamberini G, Ferioli V, Gamberini MC, Ficarra R, Tommasini S. Solid-state study of polymorphic drugs: Carbamazepine. J Pharm Biomed Anal 23:41–54, 2000.
- Hino T, Ford JL, Powell MW. Assessment of nicotinamide polymorphs by differential scanning calorimetry. Thermochimica Acta 374:85-92, 2001.
- Kawakami K, Ida Y. Application of modulated DSC to the analysis of enantiotropically related polymorphic transitions. Thermochimica Acta 427:93-99, 2005.
- Boldyreva EV, Drebushchak VA, Paukov IE, Kovalevskaya YA, Drebushchak TN. DSC and adiabatic calorimetry study of the polymorphs of paracetamol – An old problem revisited. J Therm Anal Cal 77:607-623, 2004.
- Koester LS, Mayorga P, Pereira VP, Petzhold CL, Bassani VL. Carbamazepine/beta CD/HPMC solid dispersions. II. Physical characterisation. Drug Dev Ind Pharm 29:145-15, 2003.
- Chidavaenzi OC, Buckton G, Koosha F, Pathak R. The use of thermal techniques to assess the impact of feed concentration on the amorphous content and polymorphic forms present in spray dried lactose. Int J Pharm 159:67–74, 1997.
- McGregor C, Saunders MH, Buckton G, Saklatvala RD. The use of high-speed differential scanning calorimetry (Hyper-DSCTM) to study the thermal properties of carbamazepine polymorphs. Thermochimica Acta 417:231-237, 2004.
- Gerber JJ, vanderWatt JG, Lötter AP. Physical characterisation of solid forms of cyclopenthiazide. Int J Pharm, 73:137-145, 1991.
- Li RCY, Mayer PT, Trivedi JS, Fort JJ. Polymorphism and crystallization behavior of abbott-79175, a second-generation 5-lipoxygenase inhibitor. J Pharm Sci, 85:773-780, 1996.
- Jahansouz H, Thompson KC, Brenner GS, Kaufman MJ. Investigation of the polymorphism of the angiotensin II antagonist agent MK-996. Pharm Dev Tech, 4:181-187, 1999.
- Botha SA, Guillory JK, Lötter AP. Physical characterization of solid forms of Urapidil. J Pharm Biomed Anal, 4:573-587, 1986.
- Urakami K, Beezer AE. A kinetic and thermodynamic study of seratrodast polymorphic transition by isothermal microcalorimetry. Int J Pharm 257:265–271, 2003.
- Hancock JD, Sharp JH. Method of comparing solid-state kinetic data and its application to the decomposition of kaolinite, brucite, and BaCO3. J Am Ceram Soc 55:74–77, 1972.
- There is often confusion between the meanings of the terms ‘heat’ and ‘temperature’ which can be resolved by analogy. Suppose one takes a 1L bottle of water from a fridge; it has a certain temperature (say, 6 oC) and a particular heat content (which cannot be known absolutely because the total quantity of energy in the universe is unknown – this is why changes in heat content are measured). Now, if two bottles of water had been taken from the fridge both bottles would be at the same temperature. Temperature is independent of sample quantity and is known as an intensive thermodynamic quantity. The total heat content of 2L of water, conversely, is double. Heat is an extensive thermodynamic property and is dependent upon the quantity of sample.