Nanotechnology tackles problems with noninvasive glucose monitoring
Posted: 4 May 2016 | Alfred Zehe (Universidad Autónoma de Puebla), Andreas Thomas (Medtronic GmbH), Araceli Ramírez (Universidad Autónoma de Puebla) | 2 comments
For diabetes mellitus patients who treat themselves with insulin, the need to self-monitor blood glucose in order to establish their insulin dose is a major burden, and highlights the need to develop non-invasive glucose measurement technologies. Up until now, such non-invasive systems that also have adequate measurement performance under daily life conditions have not been available. However, nanotechnology has the potential to provide a solution. Traversing all scientific disciplines its nanoscale nature means that new functionalities and properties of materials can be created, which contribute to the development of innovative products and applications. Nanomedicine extends from the medical applications of nanomaterials to nanoelectronic biosensors, and there are even possible future applications of molecular nanotechnology. Indeed, nanoparticles’ use in medicine is leaping ahead. For example, both organic and inorganic artificial nanoscale materials may be introduced into the human body and assigned diagnostic functions…
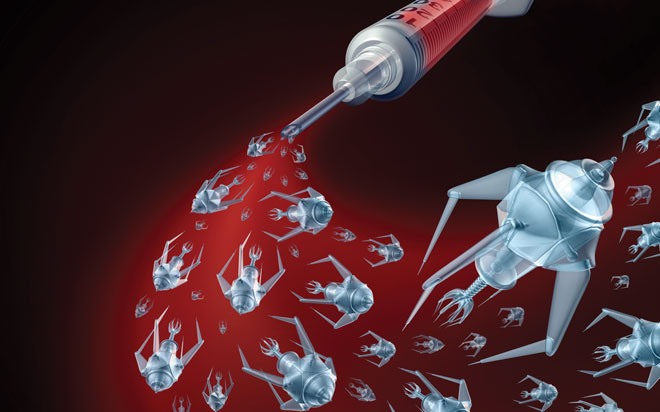
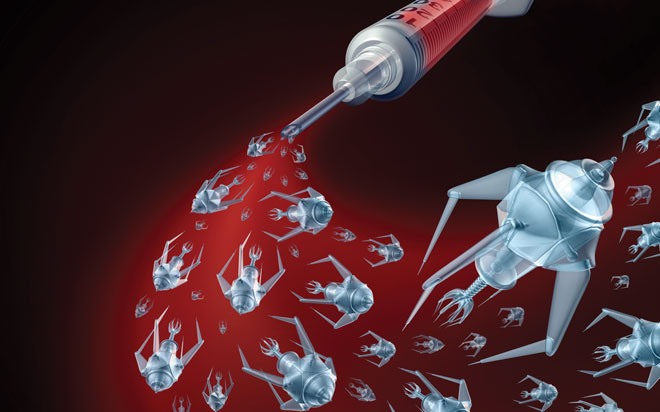
Traversing all scientific disciplines its nanoscale nature means that new functionalities and properties of materials can be created, which contribute to the development of innovative products and applications. Nanomedicine extends from the medical applications of nanomaterials to nanoelectronic biosensors, and there are even possible future applications of molecular nanotechnology. Indeed, nanoparticles’ use in medicine is leaping ahead. For example, both organic and inorganic artificial nanoscale materials may be introduced into the human body and assigned diagnostic functions1,2.
Introduction to diabetes
Diabetes is one of the most prevalent diseases in the world. According to the International Diabetes Federation (IDF), in 2015 415 million people suffered from the illness worldwide, which equates to one in eleven adults3 . And numbers continue to climb; in 2040 642 million people are anticipated to be diabetic. An important aspect of good diabetic control and successful management of the disease is the control of glucose levels, and self-monitoring blood glucose is the established method for assessing glycaemic control. Patients typically self-monitor four to six times a day in order to adapt their insulin dose to their food intake and levels of physical activity, as well as to correct for non-physiological glycaemic excursions. Since 2003 the method of continuous glucose monitoring has been available, but it is not widely established. Although it was designed to significantly reduce the necessary quantity of blood required, and lead to simpler device handling, the underlying process can still be painful. Consequently, patients desire a minimal or non-invasive measuring method.
In principle, non-invasive glucose monitoring is possible. The basis for this is the interaction of glucose molecules with applied energy (radiation, heat and electromagnetic fields, among others). A number of physical principles can be used; for example, light absorption, light scattering, polarisation of light, fluorescence, Raman scattering, and photoacoustic and impedance measurement. No sample material is required, since the applied energy field constitutes directly the measurement probe in a volume of tissue. Scientific studies have already been carried out for each of these methods4-12. However, for the measurement of glucose in a certain tissue volume, a specific interaction of the glucose molecules with the applied energy needs to take place. This is difficult because the concentration of glucose in the human body is relatively low with levels in the parts-per thousand range. Therefore, glucose signals are weak in comparison to other endogenous substances, such as water or albumin, which prevail in much higher concentrations.
The effect of signals that do not originate from glucose (‘noise’) must be compensated and separated from the true glucose signal by use of complex mathematical algorithms. A further critical consideration is that the radiated energy (e.g., a beam of light) hits a fairly complex structure when it penetrates the skin. Because of the strong anisotropy of skin and tissue, the measurement signal differs considerably, depending, for example, on the penetration depth, making it difficult to accurately measure glucose. It is therefore difficult to obtain the required accuracy for the measurement of glucose by non-invasive methods.
Nano hope
The question is: can nanotechnology provide the opportunity to develop measurement systems with the required accuracy? This complex but exciting field applies to the development and production of devices and structures with dimensions <100 nanometers and/or uses characteristic effects and phenomena in this size range (quantum effects). It opens up completely new possibilities for the development of glucose sensors and, therefore, dramatically improving the quality of diabetes patients’ lives.
In nanostructures characteristic effects take place, which are not found in bulk materials. The ratio of surface area to volume is very large. This leads to a number of physical and chemical results such as interfacial phenomena, altered reactivity, charge carrier effects and quantum mechanical changes13,14 as well as enhanced optical properties (for example quantum dot fluorescence). In miniaturised glucose sensors, such nanoscale properties can have several advantages, including higher surface areas (yielding larger currents and faster responses) and improved catalytic activities. This should result in an improved sensitivity of the glucose sensor, a lower signal-to-noise ratio and a higher selectivity of the measurement.
Nanofabrication techniques can generate glucose sensors with very small dimensions (for example by laser ablation, chemical vapour deposition (CVD) or arc discharge (nanotubes, fullerene, etc.))15. Such small sensors can be easily implanted or would be injectable as ‘glucose measurement tattoos’16,17. Additionally, they could potentially avoid the foreign body response of the immune system due to their small size and, consequently, have longer lifetimes. Finally, micro- and nanoelectronic technologies offer the possibility of cost-effective mass production. As the costs of such sensors depend massively on their widespread usage, diabetes therapy might be revolutionised if low-cost glucose sensors based on nanotechnology become available.
Examples of experimental glucose sensors
Micro- and nanoelectronics offer new solutions for glucose sensors with a high signal-to-noise ratio. Nanotubes have been well studied, especially carbon nanotubes. These can be classified as single-walled nanotubes or multi-walled ones. Typically, the diameter of a nanotube is less than one nanometer, while the tube wall can be up to 0.3 nanometers thick (Figure 1; page 00). Such molecular assemblies of carbon atoms have been presented in literature18,19. During the production process at very high temperatures, specific substances can be introduced into these nanotubes. These react with glucose (for example: glucose oxidase à bound to a fluorescent dye).
In such a way, the nanotube functions as a light amplifier: the fluorescence signal can be measured and reflects the glucose concentration. It is envisioned that such nanotubes are wrapped in a dialysis fiber and transplanted under the skin. For the glucose measurement, the skin area is irradiated with a laser and the glucose concentration measured via the fluorescent light induced. The thinner the nanotubes are, the larger the energy band gap and the greater the energy absorption and the fluorescence signal20. On the basis of single wall-nanotubes, enzyme-based optical glucose sensors were investigated21,22. In combination with enzymes for the catalysis of glucose reactions (e.g., immobilised glucose oxidase; GOx), the decisive advantage of such nanostructures is the very large surface area and the efficient electron transfer from enzyme to electrode23. Sensors of this type have a high sensitivity, linear detection range and linearity for detecting glucose. Furthermore, the carbon nanotube (CNT) fiber-based glucose biosensor was shown to be stable for up to 70 days23.
The use of nanostructured materials in glucose sensors, compared with currently-used GOx sensors of the first generation, is shown in Figure 2 (page 00)24. As with ordinary glucose sensors based on GOx, it is possible to improve electron transfer between the enzyme and the electrode by using an electrochemical mediator. This is reduced by the GOx reaction and transfers its electrons to the electrode. In this sense, an approach is to modify the nanotubes with an electrochemical mediator such as ferrocene25 or ferricyanide26. A further improvement in sensor performance is made possible by the combination of CNTs with various nanoparticles such as noble metals (silver, gold, platinum) or silica, titanium dioxide and others (Figure 2, right; page 00). This combination of nanomaterials improves the catalytic activity and the sensitivity of measurement.
Further attractive options for glucose monitoring devices are biocompatible polymeric nanosensors implanted under the skin (like a tattoo). The fluorescence properties of such sensors change in response to changes in the glucose concentration in the interstitial fluid. Such changes can be read using optical interrogation through the skin after light excitation with a laser beam. Such sensors can be based on polymeric nanosensors incorporating boronic acid derivatives to recognise glucose. Nanospheres based on N-isopropylacrylamide containing a covalently bound phenyl-boronic acid derivative, as well as two attached fluorophores, have been synthesised27. In case of a low glucose concentration, the nanospheres are small and hold the fluorophores close together. The consequence is an efficient resonance energy transfer. When there are higher glucose concentrations, the glucose binding to the boronic acid is reduced and subsequently the polymer swells, which increases the average distance between fluorophores. This therefore decreases resonance energy transfer, which increases the donor fluorescence and reduces the acceptor fluorescence. Special fluoresphores can be CNT, which demonstrate a glucose-controlled aggregation onto concanavalin A. Since the aggregates have different fluorescences than free CNTs, detection of glucose is possible through measuring the CNT fluorescence change28.
An interesting nanostructure is that of quantum dots, usually made of semiconductor material (e.g., CdSe, InGaAs or GaInP/InP). Quantum dots can be prepared by molecular beam epitaxy or lithographical processes in semiconductor layer systems (nanolayers with a few atomic layers), for example, with an electron beam and following a dry etching procedure. A quantum dot is small enough to exhibit quantum mechanical properties. Typically, it contains about 10,000 atoms. Charge carriers (electrons, holes) in the dot are so far limited in their mobility in all three spatial directions that their energy no longer continues, and only discrete values may be obtained. Quantum dots thus behave like atoms, but their shape, size or the number of electrons may be influenced. These electronic and optical properties can be tailored15, enabling production of quantum dots that have favourable optical properties for use in different sensors.
Since this sensor itself does not interact with glucose molecules directly, an attachment, for example of GOx to a quantum dot, is necessary. In this case the luminescence of the quantum dots can be quenched by hydrogen peroxide. In the presence of glucose the enzyme generates hydrogen peroxide, which quenches the quantum dots, providing an optical signal change proportional to glucose concentrations. For enhancement of glucose oxidase activity the assembling of a complex with quantum dots of different compounds, such as cadmium telluride, is possible29.
Conclusions
The ongoing miniaturisation of semiconductor integrated circuits has led to nano-technological solutions, which allow for completely new approaches to glucose sensing. At the existing technology level, it is already possible to produce glucose sensors as integrated components, such as integrated circuits. It is now a case of deciding which of the structures can be effectively produced in large quantities and could thus be inexpensive nanotechnologies, which once resolved is likely to enable the development of glucose sensors that offer a number of advantages over known CGM systems. Such sensors could be injectable under the skin and provide novel physical effects created in new nanomaterials, fabricated by specialised nanotechnologies.
References
- Thomas A. Nanotecnología, sensores y su aplicación a la medición de la glucosa en pacientes con diabetes mellitus. Nanociencia et Molectronica, 2006; Vol 4 No. 2: 682-694
- Zehe A. La Nanotecnologia como fuerza economica: Aplicaciones y Productos Mercantiles. Nanociencia et Molectronica, 2007; Vol 5 No. 2: 1015-1034
- IDF diabetes atlas. 7th edition. International Diabetes Federation 2015. http://www.diabetesatlas.org/ Last accessed January 2016
- Mattu M, Makarawicz MR, Blank TB, Lorenz AD, Monfre L, Az Evaluation of a Guideless Non-Invasive Glucose Sensor. Diabetes. 2008; 57 (Suppl. 1): A117
- Jovanovich L, Ahmann A, Edelman S, Fischer J, Garg S, Monfre S, Ruchti Human Factors Assessment for Measuring Glucose Non-Invasively. Diabetes. 2007; 56 (Suppl. 1): A9
- Combs AH, Harjunmaa HI, Kun S, Burelli RA, Keating J, Flaton K, Lock JP, Peura Optical Noninvasive Glucometer achieves ISO required clinical accuracy in pilot study. Diabetes Technology and Therapeutics. 2013; 15 (Suppl. 1): A7
- Gal A, Harman-Boehm I, Drexler A, Naidis E, Mayzel Y, Goldstein N, Horman K, Cohen S, Krasilshchikov Y. Enabling frequent blood glucose monitoring at home using a truly Non-invasive device. Diabetes Technology & Therapeutics. 2015; 17(Suppl.1):A 77
- Lyandres O, Yuen JM, Shah NC, VanDuyne RP, Walsh JT, Glucksberg Progress Toward an In Vivo Surface-Enhanced Raman Spectroscopy Glucose Sensor. Diabetes Technology and Therapeutics. 2008; 10(4): 257-265
- Lipson J, Bernhardt J, Block U, Freeman WR, Hofmeister R, Hristakeva M, Lenosky T, McNamara R, Petrasek D, Veltkamp D, Waydo S: Requirements for calibration in noninvasive Glucose monitoring by Raman J Diabetes Sci Technol. 2009; 3:233-241
- DeHennis AD, Tankiewicz S, Raisoni B, Long C, Whitehurst T, Colvin An integrated wireless Fluorimeter for a long term implantable, continuous Glucose monitoring system. Diabetes Technology and Therapeutics. 2013; 15 (Suppl. 1): A58
- Kristensen JS: Trans-Cutaneous Fluorescence Lifetime Based Continuous Glucose Reading for Long Term Diabetologia. 2005; 48 (Suppl. 1): A49
- Nielsen JK, Christiansen JS, Kristensen JS, Toft HO, Hansen LL, Aasmul S, Gregorius Clinical Evaluation of a Transcutaneous Interrogated Fluorescence Lifetime-Based Microsensor for Continuous Glucose Reading. J Diabetes Sci Technol. 2009;3(1):98-109
- Allhoff F, Lin P, Moore D. What is nanotechnology and why does it matter? From science to ethics. John Wiley and Sons Publishing House 2010. Chapter 1. The Basics of Nanotechnology: 1-19. ISBN 1-4051-7545-1
- Zehe A, Thomas Tecnología Epitaxial de Silicio, ISBN 3-8311-1438-2, www.libri.de Norderstedt 2000
- Thomas A, Torres Tapia E, Ramírez A, Zehe A. Las nanopartículas – Nanomateriales de tantas aplicaciones asombrosas en nanomedicina y nanotecnología biomédica, Internet Electron. J.Nanocs. Moletrón. 2015, 13(1): 2315-2326
- Stein EW, Grant PS, Zhu H, McShane MJ. Microscale enzymatic optical biosensors using mass transport limiting nanofilms. 1. Fabrication and characterization using glucose as a model analyte. Anal Chem. 2007; 79(4):1339-1348
- Brown JQ, McShane MJ. Modeling of spherical fluorescent glucose microsensor systems: design of enzymatic smart tattoos. Biosens Bioelectron. 2006; 21(9):1760-1769
- Radushkewitsch LK, Lukjanowitsch, VM, O struktura ugleroda, obrazujucegosja pri termitscheskom razlozenii okisi uglerodana zeleznom kontakte, J. Fis. Chim. 1952; 26: 88-95
- Iijima S, Ichihashi T, Single shell carbon nanotubes of 1nm diameter, Nature. 1993; 363: 603-605
- Strano M. The Chemistry of Single Walled Carbon Nanotubes. Applications to nanotube separation and biomolecule detection. Proceedings 2th Internationale Conference on Advanced Technology and Treatments for Diabetes 2009: O-37
- Bachilo SM, Strano MS, Kittrell C, Hauge RH, Smalley RE, Weisman RB. Structure-assigned optical spectra of single-walled carbon nanotubes. Science. 2002; 298(5602):2361-2366
- Barone PW, Strano MS. Single Walled Carbon Nanotubes as Reporters for the Optical Detection of Glucose. Journal of Diabetes Science and Technology 2009; 3(2):242-252
- Zhu Z, Song W, Burugapalli K, Moussy F, Li Y-L, Zhong X-H. Nano-yarn carbon nanotube fiber based enzymatic glucose biosensor. Nanotechnology 2010; 21(16):165501
- Cash KJ, Clark HA. Nanosensors and nanomaterials for monitoring glucose in diabetes. Trends in Molecular Medicine, December 2010; 16(12):584-593
- Qiu JD, et al. Amperometric sensor based on ferrocene-modified multiwalled carbon nanotube nanocomposites as electron mediator for the determination of glucose. Anal Biochem. 2009; 385:264–269
- Barone PW, Baik S, Heller DA, Strano MS. Near-infrared optical sensors based on single-walled carbon nanotubes. Nature Materials 2005; 4:86-92
- Zenkl G, Mayr T, Klimant I. Sugar-responsive fluorescent nanospheres. Macromol Biosci. 2008;8:146–152
- Barone PW, Strano MS. Reversible control of carbon nanotube aggregation for a glucose affinity sensor. Angew Chem Int Ed. 2006; 45:8138–8141
- Cao LH, Ye J, Tong L, Tang B. A New Route to the Considerable Enhancement of Glucose Oxidase (GOx) Activity: The Simple Assembly of a Complex from CdTe Quantum Dots and GOx, and Its Glucose Sensing. Chemistry – A European Journal. 2008; 14:9633–9640
Disclosures
Andreas Thomas is Head of Science in the Diabetes Division of Medtronic, Germany, a manufacturer and distributor of insulin pumps and glucose sensors; Araceli Ramírez is co-worker in Laboratorio de Nanotrónica, Benemerita Universidad Autonoma de Puebla, Mexico with no commercial interest in medical devices; and Alfred Zehe is Head of Laboratorio de Nanotrónica, Benemerita Universidad Autonoma de Puebla, Mexico with no commercial interest in medical devices.
Biographies
Andreas Thomas studied Physics until 1984 at the Technical University Dresden, Germany. He completed his dissertation in solid state physics in 1987 and a postdoctoral thesis in 1992. Afterwards, he worked in different fields of diabetes technology and therapeutics. He is currently Scientific Manager at Medtronic GmbH, Department of Diabetes, in Germany. Furthermore, he is interested in the development of insulin pump therapy, continuous glucose monitoring and the artificial pancreas. He works closely with his former teacher at the University of Dresden, Prof. Alfred Zehe, who heads a laboratory of nanotechnology at the University of Puebla in Mexico. He is a member of the Advisory Board in the German working group of Diabetes and Technology and was Chief Editor of the German Journal Diabetes and Technology. His address for correspondence is: Dr. Andreas Thomas, Earl-Bakken-Platz 1, 40670 Meerbusch, Germany. His email address is: [email protected].
Dr. A. Zehe is a Full Professor of Physics and Director of the Nanotechnology-Center at the Autonomous University of Puebla in Mexico. Over many years, his academic work at universities in Germany involved the progress in solid-state physics, semiconductor materials and microelectronics. His actual research fields in Mexico turn around nanomaterials and nanoelectronics in applications of life sciences and nano-medicine.
Dr. A. Ramírez teaches chemistry at the Autonomous University of Puebla in Mexico and is the leading scientist of nano-microscopy in the Laboratorio de Nanotrónica. As a collaborator of Dr. Zehe’s research group, her actual research fields involve nanomaterials and nanodevices for applications in life sciences and nano-medicine.
I would like the rest of this content.
I appreciate your atention!!!!!!
Hi Araceli, to access the full article please login or register here, thanks