Functional genomics as a tool for guiding personalised cancer treatment
Posted: 29 October 2010 |
Improved understanding of the molecular alterations in cancer cells has fuelled the development of more specific and directed cancer therapies. However, it has become clear that response rates can be low due to confounding genetic alterations that render these highly specific therapies ineffective. As a result, the costs of cancer treatment will increase enormously unless we are able to identify those patients that will benefit most from these directed therapies. In addition, it will be necessary to identify additional targets in these complex molecular networks that can be further exploited to increase overall response rates in the highly heterogenic populations of human tumours. In recent years, great expectations have been put forward for the use of functional genomic screening technologies to reach these goals.
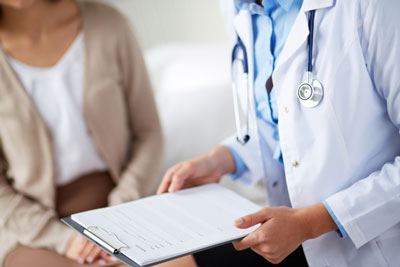
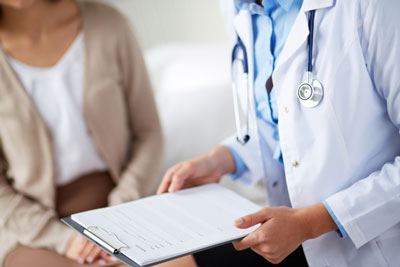
Improved understanding of the molecular alterations in cancer cells has fuelled the development of more specific and directed cancer therapies. However, it has become clear that response rates can be low due to confounding genetic alterations that render these highly specific therapies ineffective. As a result, the costs of cancer treatment will increase enormously unless we are able to identify those patients that will benefit most from these directed therapies. In addition, it will be necessary to identify additional targets in these complex molecular networks that can be further exploited to increase overall response rates in the highly heterogenic populations of human tumours. In recent years, great expectations have been put forward for the use of functional genomic screening technologies to reach these goals.
During the last few decades, great strides have been made in the development and clinical implementation of targeted therapeutics. Several successful examples exist where specific inhibition of components of signal transduction pathways controlling proliferation and survival are leading to good clinical responses. However, at the same time it has also become apparent that cancer presents itself as a very heterogeneous disease. Detailed genomic analyses and transcriptional profiling have resulted in the further distinction of several subtypes within one type of cancer. Consequently, response rates of individual tumours to pathway-targeted therapeutics can be low due to the plethora of underlying genetic alterations affecting the tumour cell’s circuitry. To improve and extend the use of targeted therapeutics, it is not only essential to identify the crucial players in these signal transduction networks but also to classify individual tumours for their specific genetic alterations correlating with an unique pathway activation profile.
One successful example of therapeutics that target specific genetic abnormalities unique for tumour cells is the kinase inhibitor Imatinib, targeting the constitutively active BCR-ABL kinase, essential for chronic myeloid leukaemia. Other successful examples include specific inhibition of EGFR and HER2 signalling in lung and breast cancer respectively, using either small molecules or monoclonal antibodies. However, by studying the different response rates in individual tumours it has also become evident that additional genetic alterations associated with (downstream) components of the signalling pathways targeted by these drugs, e.g. RAS or PI3K activation, have a strong impact on the effectiveness of these types of treatments. It is clear that not only is a detailed understanding of the molecular networks underlying cancer pathogenesis required to extend the potential targets for many cancers but also to determine the most effective treatment for each individual tumour harbouring unique genetic alterations or combinations thereof. In recent years, RNAi screening technologies have established themselves as an important strategy to identify novel targets as well as providing us with novel biomarkers that are crucial for the further development of personalised medicine.
RNAi screening strategies
The identification of novel cancer drug targets and functional biomarkers using RNAi screening tools are based on several different screening strategies. One approach is to identify novel components in important signal transduction pathways relevant for tumorigenesis based on pathway activation. The activation of a relevant pathway can be monitored by the activation of an artificial reporter gene, the induction of an endogenous target gene or the molecular consequences of pathway activation e.g. protein phosphorylation or translocation. Several cancer specific pathways including the p53, NFkB or WNT pathways have been interrogated using these different screening systems. An example of such a screen was aimed at the identification of novel modulators of the NFκB pathway with a specific focus on the enzyme class of ubiquitinspecific proteases (DUBs)1. A small shRNA library targeting 50 DUBs was used in a reporter gene assay in which NFκB DNA binding sites controlled the expression of luciferase. In this screen, CYLD, the familial cylindromatosis tumour suppressor gene, was identified as an inhibitor of NFκB signalling. The correlation between increased NFκB signalling and loss of CYLD in cylindromatosis suggested a novel therapeutic strategy. Indeed, treatment of patients suffering from cylindromatosis with salicylic acid, a known NFκB inhibitor, caused a significant decrease of small tumour lesions. This is an excellent example of how an unbiased RNAi screen led to a novel therapeutic strategy. Another example of the application of RNAi for novel target identification based on a crucial signalling pathway in colon cancer was described by Firestein et al2. In this study, two screens were performed; one aimed at the identification of regulators of ßcatenin induced transcriptional activity and one for the phenotypic consequences of ßcatenin inactivation in colon cancer cells, reduced survival. From these two screens, overlapping hits were selected and gene copy number alterations in colon cancer specimens were analysed. This combined strategy led to the identification of the gene CDK8. Indeed, inhibition of CDK8 reduces ß-catenin driven gene expression and blocks transformation, establishing CDK8 as a potential novel target for colon cancer treatment.
A major problem in the treatment of cancer is intrinsic or acquired resistance. Exposure to chemotherapeutic agents or small molecule inhibitors can select for additional (epi) genetic changes that make tumour cells insensitive to treatment. Although acquired resistance represents a major barrier in curing cancer, the increasing number of therapeutic options also requires methods that effectively determine the intrinsic sensitivity of tumours for a specific treatment. RNAi screening technologies can be used to determine functional correlations between gene expression and sensitivity to a drug. This approach is termed chemical-genetic screening and includes both drug enhancer screens and drug resistance screens. A powerful strategy for chemical genetic screens is the screening of pooled libraries consisting of vector-based shRNAs combined with barcode read-outs (Figure 1). Advantages of this method are the large numbers of vectors that can be screened simultaneously in one population exposed to the same drug concentration. In addition, it allows for the identification of genes that, upon partial knock-down, cause the resistance phenotype. This possibility is particularly important when complete knock-down of a resistance gene is lethal by itself. Finally, the drug response of the cells can be monitored over long periods of time (up to weeks), in contrast to single well assays that are mostly restricted to a short term selection (maximum five to seven days). This type of screening, named RNAi barcode screening, is illustrated by our work in which we aimed to identify genes involved in the cytotoxic response to Nutlin-3, a small molecule that inhibits the p53-MDM2 interaction3. MDM2 is a negative regulator of P53 and is frequently amplified in cancer. Inhibition of MDM2 leads to stabilisation of p53 protein. As a consequence, Nutlin-3 has strong cytotoxic effects on tumour cells expressing a wild type P53 protein but not on normal cells under physiological conditions. In this shRNA barcode screen, we identified genes encoding for proteins involved in the DNA damage response, including p53BP1. This finding led to the hypothesis that intrinsic DNA damage signalling, a hallmark of cancer cells, is essential for the activation of P53 after stabilisation by Nutlin-3. Inhibition of components of the DNA damage response makes these cells resistant to Nutlin-3. This work nicely illustrates that functional genomic screens can result in the identification of genes involved in resistance but also yielding information about the mechanism of action of novel drugs. These findings can be used for the further development and clinical testing of these new drugs in early development.
As indicated above, drug resistance screens identify genes that can be used for the reversal of drug resistance but also as predictive biomarkers that predict resistance of tumours against specific therapies. An example of such a screen is the identification of genes that confer resistance of breast cancer cells to Trastuzumab, an antibody that blocks the epidermal growth factor receptor 2, HER24. This shRNA barcode screen identified PTEN as a critical determinant for the response to Trastuzumab. Subsequent analysis of the phosphatidyl-inositol-3 kinase (PI3K)-PTEN pathway status in a group of patients treated with Trastuzumab demonstrated the clinical utility of the PI3K-PTEN pathway as a biomarker to predict Trastuzumab responses in breast cancer. Furthermore, the resulting hypothesis that inhibition of PI3K could restore the sensitivity to Trastuzumab, was demonstrated in vitro with a PI3K inhibitor combined with Trastuzumab. In the example discussed above, in-depth insight in the signalling pathway provided a means for the selection of the most relevant hits. In many other screens, this still imposes a major hurdle for the follow-up and validation. One method to rank the identified genes can be based on their tumour specific expression or mutational status. For this approach, it is important to have access to sufficiently large numbers of patient samples treated with the specific compound and of which a detailed clinical follow-up has been documented. This can pose a serious barrier in the follow-up of these large scale-screening efforts, especially when drugs in early development are studied.
Many cancers are driven by molecular events that cannot be directly targeted. This can be due to the fact that these targets elude small molecule inhibition (e.g. RAS) or that the targets are crucial for normal cells and upon inhibition would cause detrimental side effects. Therefore, alternative strategies are needed in which one aims to inhibit other targets, having a lethal effect only on tumour cells with their specific genetic alterations, and not on normal cells. The concept that a particular mutation has deleterious consequences under specific conditions is known as synthetic lethality. Two genes are defined as synthetic lethal when cells die if they have both genes mutated but can survive if either gene alone is mutated. The concept of exploring synthetic lethal gene-gene interactions for cancer therapy is very attractive as it turns a hallmark of cancer cells, specific mutations, into a weakness that can be exploited therapeutically. The classic example of a synthetic lethal interaction, although not resulting from a screening approach, is the lethal effect of inhibition of poly(ADP-ribose) polymerase (PARP) exclusively in cells carrying a mutated BRCA1 or BRCA2 gene5. However, synthetic lethal targets are not obvious and are difficult to predict or extrapolate from model organisms. In addition, it is very difficult to predict how cancer associated mutations might make tumour cells more vulnerable. The use of a genome wide RNAi approach to systematically examine the consequence of inactivation of large numbers of individual genes in human (tumour) cell lines with defined tumour specific genetic alterations could yield those novel synthetic lethal targets. The development of the shRNA barcode screening technology has been crucial for the identification of these synthetic lethal interactions. This approach allows for large numbers of screens in large panels of cell lines with relatively little work and low costs. Although most screens completed at this time lack sufficient validation in large panels of tumour cell lines to exclude context dependency or extensive in vivo validation of their identified hits, it is clear that this strategy will yield interesting candidates.
Screening model development
A continuing challenge for RNAi screening efforts remains the development of relevant screening models. Results obtained in vitro with genetically modified cell lines or panels of tumour cell lines do not necessarily recapitulate the in vivo consequences or more importantly, clinical response. Recently, several groups have embarked on RNAi screening in vivo. Although these screens are still limited by the number of genes that can be interrogated, they have yielded promising results. However, although these screens select for phenotypes that are more relevant in vivo, they do not capture the existing heterogeneity in human tumours. For this reason, a variation of this approach, direct screening of primary tumour cells isolated from a tumour sample derived from the patient, can be used. This approach, termed ‘direct functional ex-vivo screening’ has been described recently6. In this study, they interrogated the vulnerabilities of primary leukaemia cells from 30 patients with a siRNA library selectively targeting tyrosine kinases. They identified one kinase, JAK2, critical for the survival of primary leukaemia cells from 10 individual patients. Subsequently, inhibition of JAK2 kinase by a chemical compound affected the viability of the tumour cells of these individual patients in vitro. The direct functional ex vivo screening of tumour material from patients could theoretically within a few days provide vulnerabilities that can be readily applied in individually tailored cancer therapy.
Do RNAi screens live up to the expectations?
The strategies and their examples discussed above clearly illustrate the capability of RNAi screening technologies to identify novel, important insights in individual genes and the associated molecular networks that can be explored for therapeutic intervention. However, due to limitations both of a technical nature as well as the underlying biological model systems, the identified genes still require extensive validation and follow-up to establish the biological effect independent of the cellular context and under the influence of the complex in vivo environment. A first step in the validation process is the study of the correlation of the expression levels of the selected genes to either treatment response or disease outcome. Although, this does not replace the extensive follow-up, it can establish the potential relevance of the gene in a clinical setting more rapidly. At the same time, it has also become clear that the integration of different types of large scale genomic data with the results of functional genomic screens can be used to select those targets that are truly important for the biological phenotype under investigation. As the quality of these data sets increase, results of RNAi screening efforts will be easier to interpret and more valuable for the goal of the implementation of personalised medicine in cancer treatment.
References
1. Brummelkamp TR et al (2003) Nature 424(6950):797-801
2. Firestein et al (2008) Nature 455(7212):547-51
3. Brummelkamp et al. (2006) Nat Chem Biol. 2(4): 202-6
4. Berns KHM et al (2007) Cancer Cell 12(4): 395-402
5. Farmer H et al (2005) Nature 434(7035): 917-921
6. Tyner JW et al (2009) Proc Natl Acad Sci U S A 106(21):8695-8700
About the Author
Roderick Beijersbergen
Dr. Roderick Beijersbergen currently holds a position at the division of molecular carcinogenesis at the Netherlands Cancer Institute-Antoni van Leeuwenhoek Hospital (NKI-AvL) in Amsterdam (The Netherlands). He heads the NKI Robotics and Screening Center at the NKI-AvL and is part of the Cancer Systems Biology Center at the NKI-AvL. The focus of his work is the development and application of large scale functional genomic tools for high throughput screening with the aim to discover novel target for cancer therapy. In addition, his work aims to identify pathway signatures that can guide therapy decisions. Dr. Beijersbergen is credited with 32 publications in leading academic journals. He was among the first to generate large collections of vector based shRNA libraries and the application thereof has led to several new discoveries in important cancer related pathways, insights in the mechanism of drug action and potential mechanisms of drug resistance.