Article 1 Rapid microbiological methods 2011
Posted: 16 February 2011 |
This is the first in a series of articles on rapid microbiological methods that will appear in European Pharmaceutical Review during 2011. Last year, I provided an overview of rapid microbiological methods (RMMs), including validation strategies, regulatory expectations, the technical and quality benefits of RMMs as compared with conventional techniques, and an overview of a variety RMM presentations and plenary sessions during the 5th Annual PDA Global Conference on Pharmaceutical Microbiology.
During the year I also provided updates on what was new and noteworthy in the world of rapid methods, through my blog on www.rapidmicromethods.com. The response to this series was so overwhelming, I was asked to repeat the series again in 2011. Of course, I couldn’t resist (actually, my response to continue to provide guidance on RMMs was quite rapid!). As there are literally dozens of different RMM technology platforms, and just as many applications that they can be used for, it was obvious that this series would need to demystify the task of matching the right rapid method with the right application. Therefore, it is necessary to review the types of systems that are currently available, and those that are in development.
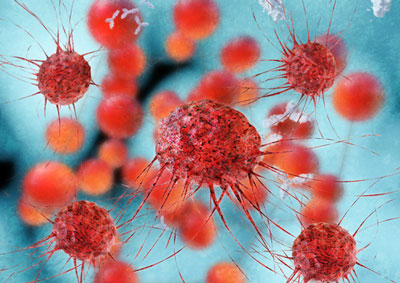
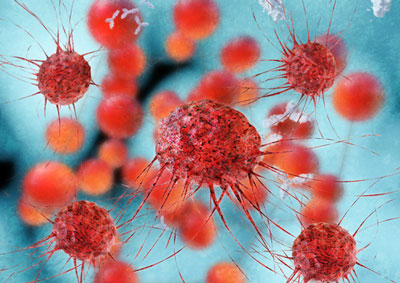
This is the first in a series of articles on rapid microbiological methods that will appear in European Pharmaceutical Review during 2011. Last year, I provided an overview of rapid microbiological methods (RMMs), including validation strategies, regulatory expectations, the technical and quality benefits of RMMs as compared with conventional techniques, and an overview of a variety RMM presentations and plenary sessions during the 5th Annual PDA Global Conference on Pharmaceutical Microbiology.
During the year I also provided updates on what was new and noteworthy in the world of rapid methods, through my blog on www.rapidmicromethods.com. The response to this series was so overwhelming, I was asked to repeat the series again in 2011. Of course, I couldn’t resist (actually, my response to continue to provide guidance on RMMs was quite rapid!). As there are literally dozens of different RMM technology platforms, and just as many applications that they can be used for, it was obvious that this series would need to demystify the task of matching the right rapid method with the right application. Therefore, it is necessary to review the types of systems that are currently available, and those that are in development.
Introduction
Rapid method technologies can detect the presence of diverse types of microorganisms or a specific microbial species, enumerate the number of microorganisms present in a sample, and can identify microbial cultures to the genus, species and sub-species levels. The manner in which microorganisms are detected, quantified or identified will be dependent on the specific technology and instrumentation employed. For example, growth-based technologies rely on the measurement of biochemical or physiological parameters that reflect the growth of microorganisms. These types of systems require the organisms in a sample to proliferate, either on a solid or liquid medium, in order to be detected and/or quantified. Viability-based systems use viability stains and/or cellular markers for the detection and quantification of microorganisms without the need for cellular growth. Artefact or cellular component-based technologies rely on the analysis of cellular components or the use of probes that are specific for microbial target sites of interest. Optical spectroscopy technologies make use of light scattering and other optical techniques to detect, enumerate and identify microorganisms. Genetic and nucleic acid amplification-based technologies may utilise PCR-DNA amplification, RNA-based transcription-mediated amplification, 16S rRNA typing, gene sequencing techniques or other novel applications. Finally, Micro-Electro- Mechanical Systems (MEMS), such as microarrays, biosensors and Lab-On-A-Chip or micro-fluidic systems offer significantly smallersized technology platforms as compared with bench-top instrumentation. Because most of us continue to use conventional agar and liquid medium for the growth of microorganisms, the best place to start is a review of growth-based rapid microbiological methods and how they might be utilised.
Growth-based RMMs
Rapid methods that employ the use of growthbased platforms are, for the most part, decreasing the time at which we can detect actively growing microorganisms. Many currently used growth-based systems continue to use conventional liquid or agar media.
As a result, the same types of applications that traditional methods are used for can also be applied to growth-based RMMs. Examples include bioburden testing, microbial limits, environmental monitoring, sterility testing and the identification or presence/absence of microorganisms. Examples of the types of core technology principles that are currently used are based on impedance microbiology, the detection of carbon dioxide (CO2), the utilisation of biochemical and carbohydrate substrates, the use of digital imaging and auto-fluorescence for the rapid detection and counting of microcolonies, fluorescent staining and enumeration of micro-colonies by laser excitation and the use of selective media for the rapid detection of specific microorganisms.
Impedance microbiology
Microbial growth results in the breakdown of larger, relatively uncharged molecules into smaller, highly charged molecules (e.g., proteins into amino acids, fats into fatty acids and polysaccharides or sugars into lactic acid). RMMs that utilise impedance employ sample holders containing incubation wells in which two electrodes are located at the bottom of each well. These systems can detect changes in measurable electrical threshold during microbial growth; for example, by monitoring the movement of ions between electrodes (conductance), or the storage of charge at the electrode surface (capacitance). Liquid media is added to each well, in addition to the sample under test, and the holder is incubated at an appropriate temperature. If microbial growth occurs, changes in impedance are detected faster than observing turbidity in the media by an operator. Usually, the levels required for detection are ~100,000 colony forming units (CFU) for bacteria and ~10,000 CFU for yeast and mould. Impedance is primarily used for the rapid detection of growth (presence/absence) and for the estimation of viable cell concentration. An example of using impedance for a semiquantitative screening tool is during preservative effectiveness testing in support of formulation development.
The detection of CO2
Microorganisms, when grown in liquid culture, produce carbon dioxide (CO2) and other metabolites. In a closed container, the amount of CO2 produced may be monitored and used as a measure of organism viability. A number of RMMs that utilise the detection of CO2 have been employed in hospital clinical labs for many years, and are used for the rapid detection of microbial contamination in blood and urine samples. Now, this type of technology is being used by the pharmaceutical industry for rapid sterility testing and other assays where the presence or absence of microbial growth is determined. Test samples are added to media bottles that contain a liquid emulsion or silicone sensor. During microbial growth, CO2 in the medium diffuses into the sensor. Hydrogen ions will then interact with the sensor resulting in a decrease in pH, and the sensor will turn colour (e.g., from gray to yellow). The rate at which CO2 is detected depends on the initial concentration of microorganisms; for example, a higher initial concentration will provide a faster detection response. However, the sensitivity level required to produce this response (i.e., a colour change in the sensor) is not known. Currently, a number of companies have received FDA-approval to use CO2 detection RMMs for rapid sterility testing of tissue and cell-based products. For one company, the 14-day sterility test was reduced to just three days.
Utilisation of biochemical and carbohydrate substrates
There are a variety of RMMs that employ a microorganism’s ability to utilise biochemical and carbohydrate substrates as sole carbon or energy sources for the rapid and automated identification of microorganisms. A suspension of a pure culture (usually from an isolated colony on an agar plate) is inoculated onto test cards or strips. Each card or strip is composed of incubation wells, and individual wells contain a single substrate in dehydrated form. The inoculated cards or strips are incubated, and if the organism under test utilises any of the substrates for cellular metabolism and growth, the turbidity, colour and/or fluorescence in the well will change. One system incorporates a dye in each carbohydrate test well, and if growth occurs, the well will turn purple. The resulting data (normally in the form of positive and negative responses) are compared with an internal database or reference library and a microbial identification is provided.
Digital imaging and auto-fluorescence of micro-colonies
During microbial growth, cells will fluoresce in the yellow-green spectral region when illuminated with blue light. Cellular autofluorescence in this spectral region is a property of all microbial cells due to the presence of ubiquitous fluorescent biomolecules including flavins, riboflavins and flavoproteins. One RMM technology utilises this phenomenon to enumerate micro-colonies developing on an agar plate in approximately one-half the time required to visualise colonies using the naked eye. Samples are filtered and the membrane is placed onto an agar cassette and incubated. During incubation, a laser excites micro-colonies to autofluoresce, which are automatically enumerated by a CCD imaging system. Particles that do not grow in size over time are ignored (via software algorithms), and because this technique is non-destructive, the agar medium can continue to incubate to obtain larger colonies that can subsequently be used for microbial identification. Companies are using this type of RMM for the rapid enumeration of microorganisms during bioburden, water, environmental monitoring, in-process, finished product and sterility testing. Fluorescent staining and laser excitation of micro-colonies A recently introduced RMM utilises fluorescent staining and laser excitation for the rapid enumeration of micro-colonies. The technology is applicable for all filterable samples, including water, in-process and finished product. A test sample is filtered and the membrane is placed onto an agar cassette. Following an appropriate incubation period, the membrane is stained with a non-fluorescent substrate. Microorganisms on the filter will take up the substrate, which is then enzymatically cleaved, liberating free fluorochrome in the microorganism cytoplasm. As the fluorochrome accumulates inside the cells, the signal is amplified. Following incubation, the membrane is placed into a reader and exposed to the excitation wavelength of the fluorescent stain. Fluorescent micro-colonies can then be counted in the instrument window or on a computer via a camera. This RMM is also non-destructive, as the membrane can be placed back onto the agar cassette and re-incubated to allow larger colonies to form that can then be used for microbial identification.
Use of selective media for the detection of specific microorganisms
Another RMM targets the presence of specific microorganisms by monitoring changes in colour or fluorescence in selective media and also detects total aerobes and yeast or mould by monitoring the generation of CO2. The system utilises disposable two-zone vials that contain an incubation zone (top of vial) for the sample and microorganism, and a reading zone (bottom of vial). The two-zones eliminate masking of the optical pathway by the product and by microbial turbidity. The time to detection depends on the initial concentration of organisms in the product sample and the threshold for bacteria is 100,000 cells/mL and 10,000 cells/mL for yeast and mould. Current test vials allow for an estimation of total aerobic count, and the detection of yeast and mould, coliforms, E. coli, lactic acid bacteria, Enterobacteriaceae, Salmonella, Pseudomonas and Staphylococcus.
Summary
Growth-based rapid microbiological methods provide opportunities for enhanced detection, enumeration and identification of microorganisms. Applications for these types of RMMs include, but are not limited to, raw material and component testing, in-process and pre-sterilisation/filtration bioburden, fermentation and cell culture monitoring, purified/process water testing, environmental monitoring, microbial limits, antimicrobial effectiveness testing and sterility testing. In my next article, we will explore the use of viabilitybased RMMs, including flow cytometry and solid phase cytometry.
About the Author
Dr. Michael J. Miller is an internationally recognised micro – biologist and subject matter expert in pharmaceutical microbiology and the design, validation and implementation of rapid micro – biological methods. He is currently the President of Microbiology Consultants, LLC (http://microbiologyconsultants.com). In this role, he is responsible for providing scientific, quality, regulatory and business solutions for the pharmaceutical industry and suppliers of new microbiology technologies. For more than 22 years, Dr. Miller has held numerous R&D, manufacturing, quality, consulting and business development leadership roles at Johnson & Johnson, Eli Lilly and Company, Bausch & Lomb and Pharmaceutical Systems, Inc. Dr. Miller has authored over 100 technical publications and presentations in the areas of rapid microbiological methods, PAT, ophthalmics, disinfection and sterilisation, is the editor of PDA’s Encyclopedia of Rapid Microbiological Methods, and is the owner of http://rapidmicromethods.com, a website dedicated to the advancement of rapid methods. He currently serves on a number of PDA’s program and publication committees and advisory boards, and is co-chairing the revision of PDA Technical Report #33: Evaluation, Validation and Implementation of New Microbiological Testing Methods. Dr. Miller holds a Ph.D. in Microbiology and Biochemistry from Georgia State University (GSU), a B.A. in Anthropology and Sociology from Hobart College, and is currently an adjunct professor at GSU. He was appointed the John Henry Hobart Fellow in Residence for Ethics and Social Justice, awarded PDA’s Distinguished Service Award and was named Microbiologist of the Year by the Institute of Validation Technology (IVT).