Aseptic preparation: The weakest link is getting stronger
Posted: 12 December 2009 | | No comments yet
The manufacture of sterile products attracts the greatest regulatory scrutiny of all product types, since manufacturing failures can be fatal and manufacturers are required to utilise the most robust sterilisation method possible to limit the probability of this occurring. However, aseptic preparation, which is the last resort manufacturing method, continues to be employed since for sensitive products this is the only feasible route. Aseptic preparation has always suffered from the potential for undetected, or undetectable, microbial ingress occurring during the manipulation of sterile components. The implementation of isolator systems have provided an advance over standard cleanroom technologies and the weakest link of aseptic preparation is getting stronger.
Microbiologically there are only two types of products, sterile and non-sterile. The latter constitutes the greatest proportion of products marketed and sold with tablets the most obvious example. Sterile products such as injections and infusions, although numerically the smaller proportion, comprise the biggest regulatory load. Annex 1 of the Rules and Guidance for Pharmaceutical Manufacturers and Distributors1 for example is the largest, when compared to medicinal gases or liquids, creams and ointments, an attribute that is understandable since the administration of non-sterile parenteral products can be fatal. Regulatory scrutiny of parenteral products was initially very limited since early injections included in British Pharmacopoeias (1874) did not require sterilisation and testing for sterility was not considered. The development of quality requirements for parenteral products has been random and primarily driven by unfortunate detrimental incidents and experiences. Pharmaceutical and medical science continues to advance and the range of drugs and devices that are now available for parenteral administration is vast, ranging from simple solutions of small molecules to monoclonal antibodies to drug eluting stents. The requirement to provide these products as sterile is absolute but there is a range of manufacturing routes to this particular quality attribute.
Sterility
The definition of sterility can at times be a variable feast depending upon the reference point employed2. For the purposes of this article the simple definition provided by Brown and Gilbert3 is useful:
“The concept of sterility is absolute. Whether or not a medicinal product is sterile is inevitably a matter of probability.”
The latter part brings into play the statistics of killing or removing organisms from a product during production or sterilisation. This is statistically dependent upon the number of organisms present at the start of the process (bioburden) and the sterilisation method and conditions employed.
Test for sterility
Since sterility is a product quality attribute, similar to drug content and early industry approaches focused on end product quality control, a test for sterility was an obvious requirement. However, sterility is not a “normal” quality attribute since micro-organisms, unlike the drug, will not be evenly distributed within a product and since the test is destructive only a small sample of a batch can be analysed. The test for sterility’s biggest failing is therefore statistical4 (STERILITY TESTING PANEL) and a product batch containing 0.1% of contaminated units or a sterility assurance level (SAL) of 1 in 1,000 has a 98% chance of passing the test for sterility. Hence, sterility cannot be “tested in” and the rational emphasis on Quality Assurance in the manufacture of this product category1.
Sterilisation
Terminal sterilisation
Currently the regulatory authorities require manufacturers to apply a sterilisation decision tree5 to decide on the sterilisation method that may be employed for a particular product. The optimum route is terminal sterilisation where the product is sealed into its final bacteria proof container and then subjected to a sterilisation process. Terminal sterilisation processes, in order of preference, utilise heat (steam or dry heat), radiation and gas (for example ethylene oxide). The regulatory requirement is that the terminal sterilisation method should be capable of providing a SAL of a one in a million chance of a contaminated container being present after sterilisation. In practice a well controlled terminal sterilisation process where the bioburden is minimal will provide a SAL well in excess of one in a million. This SAL level is beyond the detection limit of the test for sterility and terminally sterilised products are permitted parametric release based upon the measured parameters during production coupled with process validation.
Traditional sterilisation methods are however not universally applicable and multiple products cannot be processed by terminal sterilisation due to sensitivity, for example protein solutions cannot be heat treated and hydrolytically sensitive drugs have to be lyophilised. This is an increasingly prevalent feature in modern drug discovery and development. In these instances terminal sterilisation is not possible and the decision trees permit as a last resort aseptic preparation.
For liquid products sterilisation by filtration prior to aseptic preparation (see below) is often employed. In a manner similar to terminal sterilisation processes filtration sterilisation maybe quantified and assuming suitable controls of bioburden and filter performance can achieve SAL values in excess of one in a million6.
Aseptic preparation
In aseptic preparation the product is assembled from terminally sterilised (using techniques above) components with sterility maintained by the use of conditions and facilities designed to prevent microbial contamination. There is no subsequent sterilisation step post assembly. Theoretically the SAL of an aseptically prepared product should be equivalent to that of the components employed in its preparation, if no organisms are permitted to ingress. However, the assembly process always associated with aseptic preparation could lead to microbial ingress and since sterility cannot be tested in this would be undetectable, a situation that is an unavoidable Achilles heel.
Aseptic preparation and risk based quality management
A recent development in pharmaceutical manufacturing is the application of “quality risk management” within pharmaceutical quality systems7. When applied to the manufacture of sterile products this process highlights aseptic preparation8 as the area most in need of risk assessment and risk management. This conclusion is not new and even in the 1960’s9 it was recognised that aseptic manipulations, especially “exposure to air* should be kept to a minimum and operators properly attired.” Aseptic preparation has therefore always wrestled with the multiple demons10 of an absolute quality requirement (sterility), which cannot be quality controlled coupled with the unknown potential for microbial ingress via the operator and or the environment11. There is therefore a sterility assurance gap (SAG) between terminal sterilisation and aseptic preparation, which is the rationale behind sterilisation decision trees. The approach to this problem through the years has been to successively limit or reduce the potential for microbial ingress by either the operator or the environment.
Environmental control
The development of clean air technology to support the microelectronics industry has very successfully been applied to the manufacture of sterile products, through the utilisation of clean rooms and unidirectional laminar airflow (LAF) systems. The environmental quality within clean areas can be tightly controlled through the provision to the area of air passed through high efficiency particulate air (HEPA) filters, which are 99.997% efficient against 0.3µm particles12. Performance standards for clean areas have been available since the 1970’s and are present within current regulatory guidelines1. These standards are higher for aseptic processing operations compared to terminal sterilisation, which reflects the SAG between the two types of product. Of note in the current guidelines is the split, for particulate contamination, into two states “at rest”, i.e. no operators present and “in operation” i.e. operators present. In the latter state a higher level of contamination is permitted, see below.
Operator control
Despite the advances in clean air technology the human operator has remained a human operator, without any real technological or biological upgrades. As recognised above there is a requirement to properly attire the operator during aseptic processing13 and also train the operator in aseptic technique8. Despite these steps the operator and the intrusions they may make still represents the major contamination source within clean rooms and LAFs and therefore the greatest risk to product sterility.
Aseptic preparation validation
In order to prove that an aseptic manufacturing system performs to an acceptable standard validation is performed using process simulation testing, where the product is replaced with a microbiological growth medium. After manufacture and a suitable period of incubation contaminated containers should be easily detected with a quoted SAL of one in a 1,000 or 0.1%14. Note that this SAL already has a 98% chance of passing the test for sterility. Logistically it is difficult to simulate an entire production batch, which may be as high as 100,000 units and guidelines allow smaller batch sizes to be employed. In a similar fashion to the statistical limitations of the test for sterility, process simulation tests are also statistically limited if less than full production batch sizes are employed. For example, to confirm a contamination rate of 0.1% at a 95% confidence limit for a batch of 20,000 containers requires a simulation test of 4,500 containers with no more than one contaminated unit. In reality most aseptic preparation systems operate at a contamination rate lower than 0.1% (a nil failure rate is really required!), probably with batch sizes greater than 20,000 and therefore the number of containers required to statistically prove this SAL increases. The SAG therefore cannot be closed by this approach even if a full batch is simulated.
Theoretical contamination rates and environmental control
One route of microbial contamination during aseptic processing is from the environment when an organism simply falls into the product and is the drive behind the use of clean rooms and LAF’s. This again highlights the statistical nature of contamination and sterility. If it is assumed that the organisms in the environment can be treated as particles of particular sizes that settle under gravitational influence it is possible to calculate a sedimentation rate. If the concentration of organisms in the environment is known then in combination with the sedimentation rate it is possible to calculate a fallout rate at which organisms will contaminate a container of a particular neck diameter size15. The regulatory guidelines1 provide limits for the concentration of organisms in the environment at the local zone or point of fill during aseptic preparation with a current value of <1 colony forming unit (cfu)/m3. In reality most aseptic production areas will perform to a lower level of contamination, which can either be measured using active or passive air sampling methods16. If a contamination level of 0.2 cfu/m3 is assumed then a standard settle plate of 90mm diameter would have to be exposed for approximately 40 hours in a filling zone to capture one organism. This is 10 times the recommended exposure time and indicates the difficulty in conclusively measuring such low microbial contamination levels. At a microbial concentration of 0.2 cfu/m3 the contamination rate for a 500ml bottle exposed for 10 minutes would be 0.023% and a 2ml ampoule with a five second exposure during filling around 0.00001%. These rates that would easily pass the test for sterility and in combination with the statistical information on validation above again highlight the difficulty (if not impossibility) of conclusively determining a SAL for aseptic manufacture. One flaw in this argument is that it does not account for organisms “stuck into” the product by operator intervention (or fingers) a process that is operator and not environment dependent.
Isolators (see Figure 1)
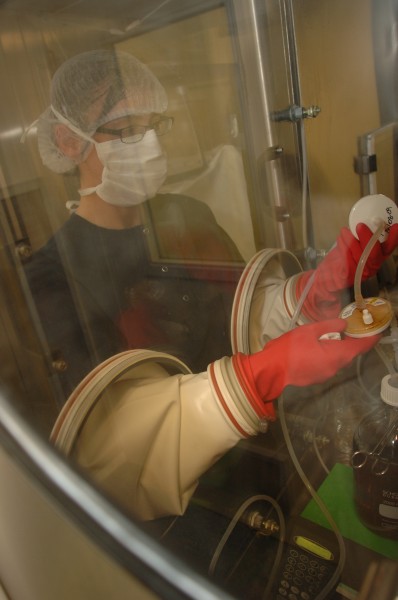
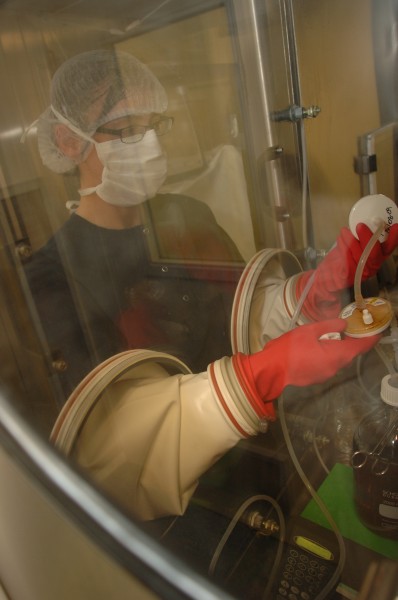
Figure 1
The requirement to provide environmental separation or defined environments can probably be attributed to the work of Schlenk (well known to chemists) who developed methods for handling air sensitive compounds. Separation or closed systems can be extended to cover operators from toxic substances or conversely sensitive systems from operators with glove boxes the most obvious iteration. Glove boxes have been applied in the nuclear industry since the 1940’s, with the requirement for containment and operator protection. However, this technology can be applied to aseptic preparation with the requirement of microbial exclusion from the point of fill zone17. Pharmaceutical manufacturing has been slow to adopt this technology and early versions were somewhat clumsy18, a feature difficult to mitigate against due to the nature of restricted access barrier systems. In essence the systems constitute a closed box or isolator with a supply (either LAF or not) and exhaust of HEPA filtered air (see above) and operator access via a barrier glove and sleeve or half-suit system. The internal surfaces of the isolator (including the sleeves, gloves and half-suits) can be sanitised (or should it be sterilised?) using gaseous systems such as vapour phase hydrogen peroxide (VHP), which can be validated to kill bioindicators (spores of 106 G. stearothermophilus) located throughout the isolator19. Entry and exit of materials (product containers, closure systems and liquids) into the isolator should be via a terminal sterilisation process (see above, which sterilises the outside as well as the inside of a container) so that the microbiological conditions inside the isolator are not compromised. In practice however, these systems do not always attain this ideal, especially the entry and exit criteria. Items not suitable for sterilisation may be transferred in via hatches after disinfection (a process not as effective as VHP), mouseholes** may be required to allow finished product exit or materials may be admitted via transfer ports. The latter producing the “ring of no confidence” since it is impossible from an engineering point of view to completely hide within the isolator, port door surfaces that have been exposed to the external environment. Even with these drawbacks isolators are an advance over clean rooms and LAF systems as the operator and the potential for contamination ingress is removed and can be further reduced by placing the isolator within a clean room to reduce the external challenge. The statistical issues mentioned above with respect to the conduct of aseptic process filling validation studies and monitoring the environment for microbial contamination have not been eliminated; in fact they have probably been exacerbated by the improvements, which will further reduce contamination rates. Various articles have placed the increase in SAL using isolators over a clean room/LAF system at between one in 10020 to one in 1,00017 or more. If clean room/LAF systems already provide a SAL of one in 1,000 or better and the improvements added by isolators are one in 1,000 or better than the SAL of aseptic preparation in isolators must be approaching one in a million or better? The SAG between terminal sterilisation and aseptic preparation is therefore diminishing.
Conclusions
There have been multiple advances over the last 50 or more years in the preparation of sterile products and the appreciation and understanding of the science behind the processes associated with this exercise. Early on in this endeavour it was recognised that aseptic preparation was the weakest link and presented a higher risk of producing a non-sterile product when compared to terminal sterilisation. In addition the inherent statistical issue of reliably counting the presence of very low number of micro-organisms further compounded and possibly confused the issue. Great effort has been expended to reduce this gap through the application of clean room/LAF systems but the biggest weakness of the presence of a human operator as a contamination source remained. The introduction of barrier systems or isolators, which remove the operator and provide a “verifiably” sterile point of fill for aseptic preparation are currently at the forefront of this process. Further technological improvements are possible but it is doubtful if they will be quantifiable using current processes and there is a need to ensure a sound scientific rationale before introduction. Despite these improvements the risks associated with parenteral therapy remain extant and it is therefore unlikely that the regulatory load will diminish. However, current systems must be performing appropriately otherwise reports of iatrogenic infection traceable to the product would be overwhelming.
The weakest link that was aseptic preparation is getting inexorably stronger.
* In fact it could be argued that even Pasteur was aware of this requirement.
** The system would be operated at an overpressure to prevent ingress through the hole!
References
- MHRA, Rules and Guidance for Pharmaceutical Manufacturers and Distributors. 2007, London: Pharmaceutical Press.
- Sharp, J., Sterile products: basic concepts and principles, in Quality in the Manufacture of Medicines and other Healthcare Products, J. Sharp, Editor. 2000, Pharmaceutical Press: London. p. 333-359.
- Brown, M.R.W. and P. Gilbert, Increasing Probability of Sterility of Medicinal Products. Journal of Pharmacy and Pharmacology, 1977. 29(9): p. 517-523.
- Halls, N.A., Sterility and sterility assurance, in Achieving sterility in medical and pharmaceutical products, N.A. Halls, Editor. 1994, Marcel Dekker: New York. p. 17-51.
- Morris, J.M., Sterilisation Decision Trees and Implementation. PDA Journal of Pharmaceutical Science and Technology, 1999. 54(1): p. 64-68.
- Dewhurst, E. and E.V. Hoxey, Sterilisation Methods, in Guide to microbiological control in pharmaceuticals, S.P. Denyer and R. Baird, Editors. 1990, Ellis Horwood Ltd: Chichester. p. 182-218.
- Anon. Quality Risk Management. 2005 [cited.
- Ahmed, R., et al., PDA survey of quality risk management practices in the pharmaceutical, devices, & biotechnology industries. PDA J Pharm Sci Technol, 2008. 62(1): p. 1-21.
- Whittet, T.D., Sterile Products, in Sterilisation and Disinfection, T.D. Whittet, W.B. Hugo, and G.R. Wikinson, Editors. 1965, William Heinemann Medical Books: London. p. 5-123.
- Akers, J.E. and J.P. Agalloco, Aseptic processing, elephants, blind men, and sterility. PDA J Pharm Sci Technol, 2002. 56(5): p. 231-4.
- Whyte, W., et al., An Evaluation of the Routes of Bacterial Contamination Occurring During Aseptic Pharmaceutical Manufacturing. Journal of Parenteral Science and Technology, 1982. 36(3): p. 102-108.
- White, P.J.P., The design of controlled environments, in Guide to microbiological control in pharmaceuticals, S.P. Denyer and R. Baird, Editors. 1990, Ellis Horwood Ltd: Chichester. p. 87-124.
- Reinmuller, B. and B. Ljungqvist, Modern cleanroom clothing systems: People as a contamination source. Pda Journal of Pharmaceutical Science and Technology, 2003. 57(2): p. 114-125.
- Prout, G., The use of process simulation tests in the evaluation of processes for the manufacture of sterile products. 1993, Swindon: The Parenteral Society.
- Whyte, W., Sterility Assurance and Models for Assessing Airborne Bacterial Contamination. Journal of Parenteral Science and Technology, 1986. 40(5): p. 188-197.
- Andon, B.M., Active air vs. passive air (settle plate) monitoring in routine environmental monitoring programs. PDA J Pharm Sci Technol, 2006. 60(6): p. 350-5.
- Coles. T., Isolation Technology: A practical guide. 1998, Buffalo Grove: Interpharm Press.
- Farquaharson, G. and W. Whyte, Isolators and barrier devices in pharmaceutical manufacturing. Pda Journal of Pharmaceutical Science and Technology, 2000. 54(1): p. 33-43.
- Mau, T., et al., Development of a sterilizing in-place application for a production machine using vaporized hydrogen peroxide. Pda Journal of Pharmaceutical Science and Technology, 2004. 58(3): p. 130-146.
- Agalloco, J., et al., Risk management, cGMP, and the evolution of aseptic processing technology. PDA J Pharm Sci Technol, 2009. 63(1): p. 8-10.