Continuous biomanufacturing – where are we now?
Posted: 28 October 2021 | Hannah Balfour (European Pharmaceutical Review) | No comments yet
The biopharmaceutical industry is driven by the need to increase production and reduce costs, while maintaining product quality – and has increasingly been focused on intensification of processes to accomplish this goal. In this article, European Pharmaceutical Review’s Hannah Balfour summarises the potential benefits of implementing continuous bioprocessing and manufacturing as a method of process intensification, the current state of continuous process implementation and some ongoing challenges.
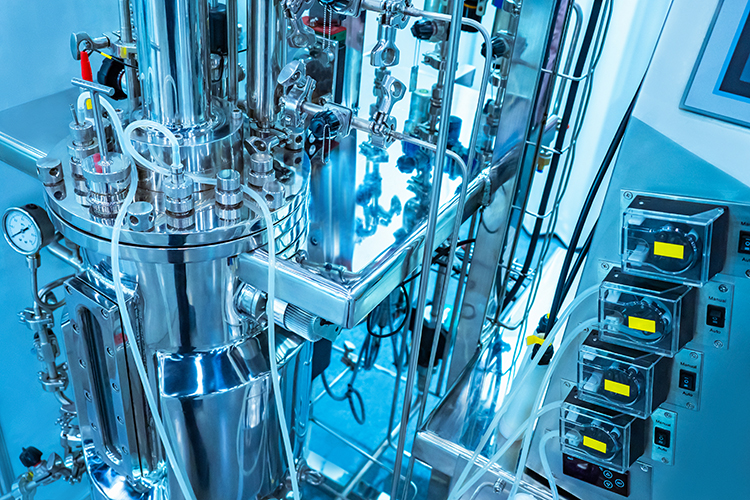
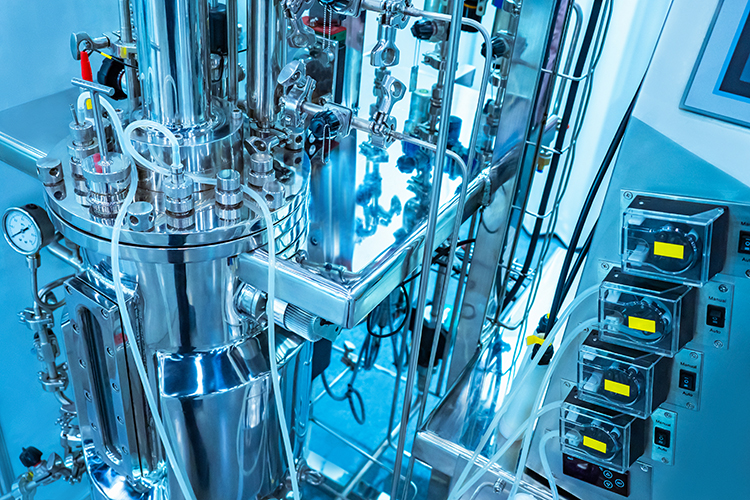
BIOPHARMACEUTICALS are a $331 billion industry,1 known for their precision, innovativeness and life-changing potential; however, stringent regulations and complex bioproduction methods that result in high manufacturing and product costs continue to limit the growth of this otherwise thriving sector.
To cut expenditure and complexity, as well as enhance process efficiency for large-scale biologics manufacturing, the industry is investing in the R&D of intensified bioprocesses. One such method under development is continuous biomanufacturing – the production of finished biopharmaceuticals using an uninterrupted process, consisting of an integrated sequence of more than one unit operation.
Continuous bioprocessing is a single unit operation within biomanufacturing where raw materials are continuously loaded, processed and unloaded. This market was valued at just $82 million in 2020 but is expected to quadruple over the next few years, growing to almost $350 million by 2027 at an anticipated 23 percent compound annual growth rate (CAGR).2 Market research suggests the major factors encouraging this growth include the rising demand for biopharmaceuticals, technological developments in continuous bioprocessing, and various initiatives supporting its adoption, as well as the shift towards digitalisation and automation driven by Pharma 4.0.2
Why develop and implement continuous manufacturing?
The biopharmaceuticals sector is inherently complex. While the rising adoption of personalised therapies, such as autologous cell therapies, calls for extremely small batches and manufacturing flexibility, increased demand for allogeneic products, therapeutic proteins such as antibodies and vaccines conversely necessitates large-scale production of quality products. While batch is a well-established manufacturing doctrine that provides process flexibility and requires less precise and robust controls, it is somewhat inefficient for the larger-scale production requirements of the modern-day biopharmaceutical industry, since it enhances complexity and thus costs. Continuous manufacturing, however, offers the benefit of streamlined processes and a reduced manufacturing facility footprint to produce vast volumes of low-cost, high-quality biopharmaceuticals. The potential benefits of continuous manufacturing are too numerous for complete examination, so here I review two of the key ones: process efficiency and cost, and product quality.
The continuous manufacturing price tag
The economic benefits of continuous manufacturing include reduced capital expenditures, smaller facility footprints and lower overall cost of goods.3 This has been confirmed in various studies and reviews – the key findings of several are outlined below
The economic benefits of continuous manufacturing include reduced capital expenditures, smaller facility footprints and lower overall cost of goods”
In a paper evaluating the cost of goods resulting from continuous manufacturing for liposomal formulations, Worsham et al. found that the continuous process produced 1,053 filled units/h, where the batch process only produced 125 units/h. This was an “8.4-fold increase in output for the same overhead costs based on units per hour and a 24-fold output increase for the same process preparation costs and single-use componentry costs.”3 However, they noted that this does not take into account costs needed to implement a continuous manufacturing setup. In terms of monoclonal antibody (mAb) production, Mahal et al. compared costs for single‑use and stainless-steel batch, single-use continuous and single-use hybrid (combining batch and continuous elements) bioprocessing setups across commercial scales of mAb production from 100 to 3,000 kg/year. They found that both single-use batch and continuous setups had an approximately 35 percent advantage in terms of cost of goods over stainless steel batch at 100 kg/year. Beyond production of 500 kg/year, single-use batch became less favourable, and from 1,000 kg/year the continuous facilities became at least as cost effective as stainless-steel batch, if not more (nine percent advantage at 3,000 kg/ year).4 They concluded that across the whole project lifecycle, end-to-end continuous facilities become more cost effective than stainless-steel batch facilities when accounting for costs from both drug development and commercial activities.
Quality benefits
- Smaller equipment and manufacturing footprint
- Reduced waste
- Potential for real-time release of products
- Faster time-to-market with reduced holding and processing times.
For more detail, a broad examination of the benefits was published by Chong Hock et al. in the Generics and Biosimilars Initiative Journal earlier this year.6
Which continuous bioprocessing technologies are currently in use?
Despite the evident benefits, the application of continuous processing – especially in full end-to-end continuous manufacturing setups – remains limited in the biopharmaceutical industry. In terms of application, continuous upstream processes are more established, with perfusion technologies having been on the market for decades and approximately 20 US Food and Drug Administration (FDA)-approved biologics currently manufactured using this process.7 The majority of these are antibodies and enzymes.
Despite the evident benefits, the application of continuous processing – especially in full end-to-end continuous manufacturing setups – remains limited in the biopharmaceutical industry”
Perfusion processes keep cell counts constant while the culture supernatants are refreshed at regular intervals, allowing for large product volumes to accumulate. In recent years, advances have enabled some systems to support 10-fold higher cell concentrations, over 100 × 106 cells/ml,8 and thus generate higher product titers. However, for the most part, culture media and additives preparation is still done in batches, and cumulative sales of leading alternating tangential flow (ATF) perfusion systems are relatively low after 15 years.9 The implementation of continuous downstream processes has lagged somewhat. This is primarily because it is more challenging to operate in this manner, since each downstream bioprocessing step uses only a fraction of the material from the step before, as the product becomes increasingly refined. However, some continuous chromatography solutions such as countercurrent chromatography have entered the market. These overcome the step issue by spatially separating the various processes (washing, elution, etc) into individual chromatography columns.7 In 2020, the 17th Annual Report and Summary of Biopharmaceutical Manufacturing Capacity and Production reported that 44.2 percent of biopharma professionals were evaluating upstream continuous processing or perfusion technologies and 40 percent downstream continuous purification or chromatography systems.10 The authors found that biomanufacturers were leading in terms of investigating continuous upstream bioprocessing, while contract manufacturing organisations (CMOs) led the charge on downstream process investigations. The two were almost equally matched (38.1 and 40 percent, respectively) in terms of their investigation of automating process controls and monitoring.10
Room for future development
To capitalise on the potential of continuous manufacturing, there are certain challenges that must be overcome, including clarification of regulations and facilitating flexibility, while also balancing environmental sustainability.11 The ICH recently released its draft Q13 guideline on Continuous Manufacturing of Drug Substances and Drug Products12 for public consultation. This guidance is anticipated to come into effect in late 2022 or early 2023 and provides “scientific and regulatory considerations for the development, implementation, operation and lifecycle management of continuous manufacturing”. The guidance is specifically aimed at small organic molecules and therapeutic proteins, yet may also be applicable to larger biological molecules.
With the publication of ICH Q13 on the horizon, and other regulatory efforts to encourage continuous biomanufacturing, the future seems bright for this exciting production approach”
Flexibility is essential in biopharmaceutical manufacturing, given the ever-changing supply demands for products. Flexibility enables manufacturers to cope with changes in need, such as the COVID-19 pandemic demand surges, or more typical changes to clinical trials or clinical adoption timelines. However, continuous bioprocessing facilities are not only found to be more efficient in single-product, high-volume manufacturing runs, but are also often made specifically to manufacture a single product, rather than adapt for different processes.11 These both limit flexibility; thus, to overcome them, R&D efforts are underway to intensify processes and create modular biomanufacturing platforms. Additionally, the implementation of single-use systems has been used to improve flexibility; allowing bioreactors, chromatography columns and filters to be disposed of after each run and enabling a simpler shift to a new product or process.11 However, there are some limitations, including their unsuitability for very high cell densities and substantial environmental impact.11 In terms of environmental sustainability, experts state that appropriate waste disposal procedures are crucial to adopting these systems and that, if done correctly, continuous bioprocessing could actually improve sustainability by requiring a smaller setup, limiting wastage, enhancing productivity and efficiency, and enabling automation.11
Final thoughts
The potentially transformative effect continuous bioprocessing and manufacturing could have on the biopharmaceutical industry is immense. Research has shown that end-to-end continuous bioprocessing could reduce cost of goods by 30 percent, minimise intra-product heterogeneity and increase quality, all within a smaller setup. However, to capitalise on these potential benefits, regulatory clarity and other technical considerations, including enhanced process flexibility, must be addressed. With the publication of ICH Q13 on the horizon, and other regulatory efforts to encourage continuous biomanufacturing, the future seems bright for this exciting production approach.
About the author
Hannah Balfour is the Assistant Editor of European Pharmaceutical Review
References
- Infogence Global Research. Global Biopharmaceutical Market (2021-2026) by Product Type, Therapeutic Application, Geography, Competitive Analysis and the Impact of Covid-19 with Ansoff Analysis [Internet]. Infogence Global Research; 2021. Available from: https://www.researchandmarkets.com/reports/…
- Meticulous Market Research Pvt. Ltd. Continuous Bioprocessing Market by Product (Filtration, Chromatography, Centrifuges, Consumables), Application (Commercial {Vaccines, Monoclonal Antibodies}, R&D), End User (Pharmaceuticals, Biotechnology, CROs), and Geography – Forecast to 2028 [Internet]. 2021. Available from: https://www.researchandmarkets.com/reports/5337908…
- Worsham R, Thomas V, Farid S. Potential of Continuous Manufacturing for Liposomal Drug Products. Biotechnology Journal. 2018;14(2):1700740.
- Mahal H, Branton H, Farid S. End‐to‐end continuous bioprocessing: Impact on facility design, cost of goods, and cost of development for monoclonal antibodies. Biotechnology and Bioengineering. 2021;118(9):3468-3485.
- Walther J, Lu J, Hollenbach M, et al. Perfusion Cell Culture Decreases Process and Product Heterogeneity in a Head‐to‐Head Comparison With Fed‐Batch. Biotechnology Journal. 2018;14(2):1700733.
- Hock S, Siang T, Wah C. Continuous manufacturing versus batch manufacturing: benefits, opportunities and challenges for manufacturers and regulators. Generics and Biosimilars Initiative Journal. 2021;10(1):44-56.
- Schmidt S. Drivers, Opportunities, and Limits of Continuous Processing – BioProcess International [Internet]. BioProcess International. 2017 [cited 13 September 2021]. Available from: https://bioprocessintl.com/manufacturing/continuous-bioprocessing…
- Clincke M, Mölleryd C, Zhang Y, et al. Very high density of CHO cells in perfusion by ATF or TFF in WAVE bioreactor™. Part I. Effect of the cell density on the process. Biotechnology Progress. 2013;29(3):754-767.
- Langer E. Biomanufacturing: Demand for Continuous Bioprocessing Increasing – Bioprocess Development Forum [Internet]. Processdevelopmentforum.com. 2020 [cited 13 September 2021]. Available from: http://www.processdevelopmentforum.com/articles/biomanufacturing…
- TOP 15 TRENDS IN BIOPHARMACEUTICAL MANUFACTURING, 2020 [Internet]. 17th ed. Rockville, Maryland: BioPlan Associates, Inc.; 2020 [cited 13 September 2021]. Available from: http://www.biopharma.com/TRENDS.pdf
- Kumar A, Udugama I, Gargalo C, Gernaey K. Why Is Batch Processing Still Dominating the Biologics Landscape? Towards an Integrated Continuous Bioprocessing Alternative. Processes. 2020;8(12):1641.
- CONTINUOUS MANUFACTURING OF DRUG SUBSTANCES AND DRUG PRODUCTS Q13 [Internet]. International Council for Harmonisation of Technical Requirements for Pharmaceuticals for Human Use (ICH); 2021 [cited 13 September 2021]. Available from: https://database.ich.org/sites/default/…
Issue
Related topics
Biologics, Biopharmaceuticals, Bioprocessing, Bioproduction, Drug Manufacturing, Industry Insight, Process Analytical Technologies (PAT), Sustainability, Technology, Therapeutics
Related organisations
International Conference on Harmonisation (ICH), Merck, US Food and Drug Administration (FDA)