What to consider when formulating bacteriophages
Posted: 22 November 2021 | Carolina Moraes de Souza, Dr Michael Koeris | No comments yet
In this article, Dr Michael Koeris, Associate Professor, Bioprocessing at Keck Graduate Institute, and Carolina Moraes de Souza, Keck MEng graduate, now Process Development Senior Associate at Amgen, discuss the key formulation considerations for bacteriophages, a potential solution to antimicrobial resistance, and why process development should be an early consideration.
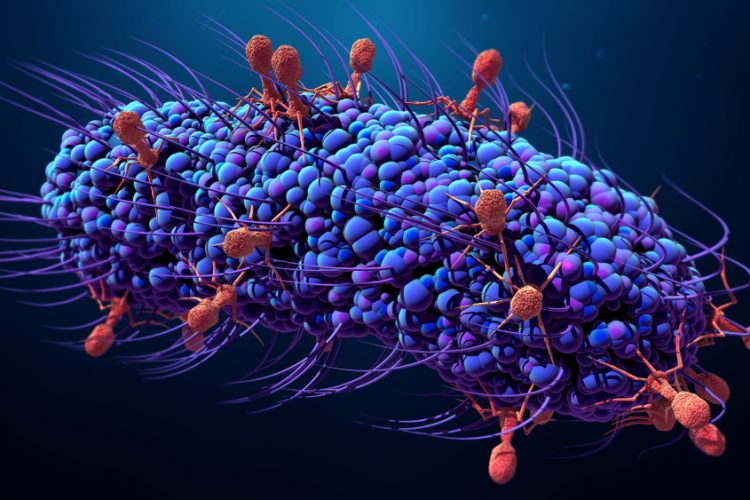
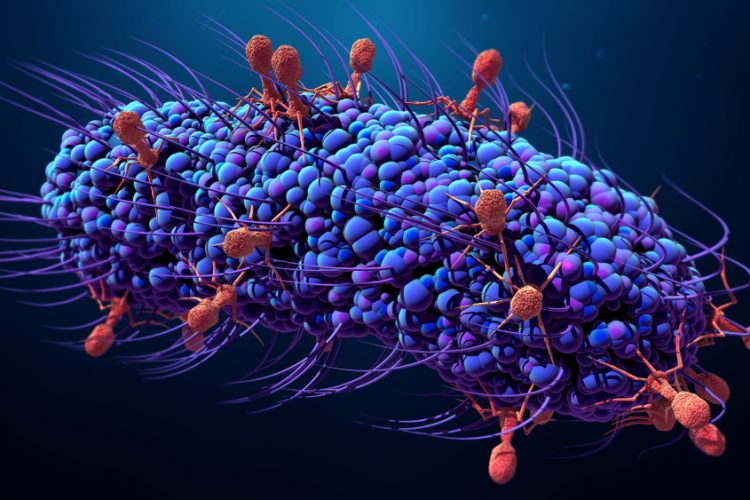
The menace of multidrug-resistant (MDR) bacteria has been growing every year, calling for new approaches to combat the lethal infections they cause. Bacteriophages, a group of viruses discovered over 100 years ago, have re-emerged as a subject of interest, drawing significant attention from the biotechnology industry. Despite being obscured by the practicality and broad spectrum of antimicrobials in the 1940s, these viruses have a unique characteristic that most antimicrobials lack: the ability to target specific bacteria, thus preserving the native microbiome.
This particular attribute also makes bacteriophages interesting prospects for other applications, such as microbiome regulation, which has been linked to several autoimmune disorders that affect both the gut and the skin.1-4 Additionally, engineered phages invite an even broader spectrum of applications, with potential for use as a delivery system for engineered proteins5 and to help modulate the tumour microenvironment for mucosal cancers, such as colorectal cancer.6 Moreover, personalised phage therapies would have still greater value for their ability to be tailored to each patient.7 They can also act in a synergistic manner with traditional antimicrobials by targeting bacterial resistance mechanisms from the inside.
Bacteriophages have already proven their utility in the drug discovery space, through what is known as phage display,8 and have recently been making a comeback in the therapeutic space. As of October 2021, there are approximately 20 clinical trials recruiting patients with a phage therapy as intervention, one of which is Phase III. This comeback is a result of the tremendous advances that have taken place in the biotechnology industry. The field, which in the last 40 years has been growing – driven by innovation and under strict regulation – has accumulated a breadth of knowledge on how to manufacture biological entities. One hundred years ago, when phages were discovered, knowledge on how to mass produce biological entities was limited. However, as the pool of tools at our disposal expanded, it became far more feasible to bring phage therapies to the clinic.
Nonetheless, developing a phage-based product is not an easy task and requires careful deliberation, as phages have unique characteristics and must overcome numerous challenges imposed by regulators.9,10 On top of the challenges posed by drug substance manufacturing, the formulation stage is particularly critical. Despite being one of the last stages in the manufacturing process, it should be carefully considered early on.
Formulation selection
The first step in drug development is designing a target product profile (TPP), which is a document detailing its purpose and use. One particularly important decision to make about the drug relates to its dosage form.11 Is it liquid, solid or semi-solid? Does it require a special coating? Is delayed delivery desired? Will it be part of a device? Additionally, there are several factors that are important to assess when designing the formula and the process for formulation, independent of the formulation type. Unique to the case of phages, some factors appear to play an important role. These fall into two categories – process-related and formulation-related factors – and are enumerated in Table 1.
Table 1. Process- and formulation-related factors that can impact phage infectivity.12-15
Process related | Formulation related |
Adsorption | pH |
Shear stress | Ionic strength |
Process temperature | Storage temperature |
Exposure to high frequencies |
|
Furthermore, choosing the right excipients requires a deep understanding of the physicochemical properties of bacteriophages. Each bacteriophage has unique characteristics that must be preserved in order to maintain their infectivity. Table 2 compiles several formulations that were tested by different groups for different phages.
Table 2. Formulations for phage solutions across multiple types. A few common elements include buffering agents and cryopreservants.
Phage | Formulation | Type | Reference |
PEV2 | Salt-magnesium buffer (SMB) 5.2 g/L, sodium chloride 2 g/L, magnesium sulfate 6.35 g/L, Tris-HCL 1.18 g/L, Tris base 0.01 percent gelatin solution with pH adjusted to 7.5, D-(+)-Trehalose dihydrate (60 percent and 40 percent w/v), mannitol (20 percent and 40 percent w/v) L-leucine (20 percent w/v). | Solid | Leung 2016(16) |
UAB_Phi20, UAB_Phi78, or UAB_Ph87 | 3.2 percent w/v trehalose, MgSO4 (10mM, pH 6.1), lipid mixture of 1,2-dilauroyl-racglycero-3-phosphocholine (DLPC), cholesteryl polyethylene glycol 600 sebacate (Chol-PEG600), cholesterol (Chol), and cholesteryl 3-N-(dimethylaminoethyl) carbamate hydrochloride (cholesteryl) (1:0.1:0.2:0.7 molar ratio). | Solid -Liposome Encapsulated | Colom 2015(17) |
KOX1 | Phage concentrated in PBS. Suppository composed by gelatin powder, purified water and Glycerol. Final concentration at 4.5 ⇥ 108 PFU/g. | Solid – Suppository | Brown 2017(18) |
phiIPLA35, phiIPLA88, phiIPLA-RODI and phiIPLA-C1C | SM buffer (20 mM Tris HCl, 10 mM MgSO4, 10 mM Ca(NO3)2 and 0.1 M NaCl, pH 7.5), 0.8 M trehalose, 0.8 M sucrose, 15 percent glycerol or 11 percent skim milk, final titer ranging from 108 to 109 PFU/mL. | Liquid | Gonzalez-Menendez 2018(19) |
Something that can be challenging is designing a product that consists of a cocktail of multiple phages. For example, it is important to pay close attention to the charge of the phage/phages in question, as it could influence mixing/aggregation and could impact interactions with the excipients.20 Aggregation can be detrimental, as it can damage the tail fibres, invalidating the phage infectivity.21
Solid formulations
This is one of the most commonly used formulations for traditional pharmaceuticals and there is extensive literature on the subject. Nonetheless, this type of formulation is uncommon for biopharmaceuticals and consequently it is important to consider the use of coating or encapsulation to avoid degradation in the gastrointestinal (GI) tract.19 Numerous authors have investigated encapsulating bacteriophages in polymers, such as alginate, as well as in liposomes.19,22 Overall, the use of encapsulation techniques can improve delivery, as well as reduce losses in yield during drying processes.
Nonetheless, solid formulations are not limited to pills as they also include devices. For example, catheters and bandages can have the drug adsorbed in their surface, which offers another promising application for phage therapy.
Liquid formulations
When compared to the other two types of formulations, liquid formulations are the simplest.
A caveat to this simplicity is when the desired use targets the respiratory and GI tract. Unfortunately, many excipients commonly used in liquid formulations are not safe for use in the respiratory tract.15 and the harsh environment encountered in the stomach and gut calls for special protection.19 One possible solution could be encapsulation, as mentioned above.
Semi-solid formulations
This type of formulation substantially expands the potential application of phage therapies, from acne to pulmonary infections.23 Nonetheless, this particular type of formulation requires careful assessment due to its diversity. The first consideration regards the type of semi-solid formulation; for example, creams, gels and ointments have different ratios between polar and non-polar excipients, which could impact solubility as phages are polar in nature.24 Still on the choice of excipients, in the case of phage therapies it seems important to avoid ionic bases, as these can interact with the pages and impact their infectivity.23
Additionally, the selected excipients must take account of the following:
- Target tissue
- Levels of permeation required
- Irritation
- Compatibility with container-closure system
- Active pharmaceutical ingredient (API) stability.
Finally, the mixing process can also present challenges, as semi-solid formulations are often more viscous than liquid formulations. Highly viscous fluids tend to offer high shear stress, which can impact phage infectivity.25,26
Scale-up
…to bring a phage therapy product to market, it is paramount to have a deep understanding of the whole picture, as each step of the manufacturing process has its own challenges”
After designing the desired formulation and optimising its parameters to meet optimal phage stability, one more challenge arises: the formulation process scale. This prospect addresses how to properly scale the process to meet product demand, while maintaining yield and speed. Most of the time for biologics, and the same applies to phages, challenges tend to appear within the drying process.27 The commonly used freeze-drying process is complicated and involves high complexity heat and mass transfer, which makes the process hard to model. A few factors that are key in this process include: load size, chamber pressure, ramp rate and shelf temperature.28 A systematised approach is needed, but so far there is no well-defined framework in place for drying processes.27 A new alternative that has been gaining attention is the use of spray-drying. This technology offers several advantages over the traditional approach, the main ones being its scalability and mode of operation (continuous versus batch). Nonetheless, attention must be paid to the process parameters to avoid protein degradation and losses in yield. As noted by Leung et al.,16 spray drying can cause yield losses as the process can expose phages to high frequencies.
Conclusion
This short paper focused on formulation considerations for bacteriophages. Nonetheless, to bring a phage therapy product to market, it is paramount to have a deep understanding of the whole picture, as each step of the manufacturing process has its own challenges. In addition, analytical and quality control are also critical elements to successfully meet regulators’ requirements and expectations. Therefore, it is the opinion of the authors, that process development should begin early in the drug development process, given its impact on the final product.
About the authors
Dr Michael Koeris is an Associate Professor in Bioprocessing at Keck Graduate Institute.
Carolina Moraes de Souza is a Keck MEng graduate and now Process Development Senior Associate at Amgen.
References
- Wang BSH, Chan MPHH, Ni MDMY, et al. Bacteriophage of the Skin Microbiome in Patients with Psoriasis and Healthy Family Controls. J Invest Dermatol. 2019;1–39.
- Belleghem JDV, Dąbrowska K, Vaneechoutte M, et al. Interactions between Bacteriophage, Bacteria, and the Mammalian Immune System. Viruses. 2018;11(1):10.
- Kelly CJ, Zheng L, Campbell EL, et al. Crosstalk between Microbiota-Derived Short-Chain Fatty Acids and Intestinal Epithelial HIF Augments Tissue Barrier Function. Cell Host Microbe. 2015;17(5):662–71.
- Międzybrodzki R, Borysowski J, Kłak M, et al. In Vivo Studies on the Influence of Bacteriophage Preparations on the Autoimmune Inflammatory Process. BioMed Res Int. 2017;2017:1–9.
- Pires DP, Cleto S, Sillankorva S, et al. Genetically Engineered Phages: a Review of Advances over the Last Decade. Microbiol Mol Biol Rev. 2016 Sep;80(3):523–43.
- Zheng D-W, Dong X, Pan P, et al. Phage-guided modulation of the gut microbiota of mouse models of colorectal cancer augments their responses to chemotherapy. Nat Biomed Eng. 2019;1–15.
- Tan X, Chen H, Zhang M, et al. Clinical Experience of Personalized Phage Therapy Against Carbapenem-Resistant Acinetobacter baumannii Lung Infection in a Patient With Chronic Obstructive Pulmonary Disease. Front Cell Infect Microbiol. 2021 Feb 26;11:631585.
- Hess KL, Jewell CM. Phage display as a tool for vaccine and immunotherapy development. Bioeng Transl Med. 2019;5(1):e10142.
- Fauconnier A. Phage Therapy Regulation: From Night to Dawn. Viruses [Internet]. 2019 Apr 17 [cited 2021 May 2];11(4). Available from: https://www.ncbi.nlm.nih.gov/pmc/articles/PMC6521264/
- Pirnay J-P, Merabishvili M, Van Raemdonck H, et al. Bacteriophage Production in Compliance with Regulatory Requirements. In: Bacteriophage Therapy [Internet]. New York, NY: Springer New York; 2018 [cited 2021 Jan 17]. p. 233–52. (Methods in Molecular Biology; vol. 1693). Available from: http://link.springer.com/10.1007/978-1-4939-7395-8_18
- Lambert WJ. Considerations in Developing a Target Product Profile for Parenteral Pharmaceutical Products. AAPS PharmSciTech. 2010 Sep;11(3):1476–81.
- Malik DJ, Sokolov IJ, Vinner GK, et al. Formulation, stabilisation and encapsulation of bacteriophage for phage therapy. Adv Colloid Interface Sci. 2017;249:100–33.
- Cortés P, Cano-Sarabia M, Colom J, et al. Nano/Micro Formulations for Bacteriophage Delivery. In: Bacteriophage Therapy [Internet]. New York, NY: Springer New York; 2018 [cited 2021 May 16]. p. 271–83. (Methods in Molecular Biology; vol. 1693). Available from: http://link.springer.com/10.1007/978-1-4939-7395-8_20
- Matinkhoo S, Lynch KH, Dennis JJ, et al. Spray-dried Respirable Powders Containing Bacteriophages for the Treatment of Pulmonary Infections. J Pharm Sci. 2011 Dec;100(12):5197–205.
- Chang RYK, Wallin M, Lin Y, et al. Phage therapy for respiratory infections. Adv Drug Deliv Rev. 2018 Aug;133:76–86.
- Leung SSY, Parumasivam T, Gao FG, et al. Production of Inhalation Phage Powders Using Spray Freeze Drying and Spray Drying Techniques for Treatment of Respiratory Infections. Pharm Res. 2016 Jun;33(6):1486–96.
- Colom J, Cano-Sarabia M, Otero J, et al. Liposome-Encapsulated Bacteriophages for Enhanced Oral Phage Therapy against Salmonella spp. Pettinari MJ, editor. Appl Environ Microbiol. 2015 Jul 15;81(14):4841–9.
- Brown TL, Petrovski S, Hoyle D, et al. Characterization and formulation into solid dosage forms of a novel bacteriophage lytic against Klebsiella oxytoca. Schuch R, editor. PLOS ONE. 2017 Aug 17;12(8):e0183510.
- González-Menéndez E, Fernández L, Gutiérrez D, et al. Comparative analysis of different preservation techniques for the storage of Staphylococcus phages aimed for the industrial development of phage-based antimicrobial products. Dąbrowska K, editor. PLOS ONE. 2018 Oct 11;13(10):e0205728.
- Merril CR, Biswas B, Carlton R, et al. Long-circulating bacteriophage as antibacterial agents. Proc Natl Acad Sci. 1996 Apr 16;93(8):3188–92.
- Yehl K. Engineering Phage Host-Range and Suppressing Bacterial Resistance through Phage Tail Fiber Mutagenesis. :21.
- Ball RL, Bajaj P, Whitehead KA. Oral delivery of siRNA lipid nanoparticles: Fate in the GI tract. Sci Rep. 2018 Dec;8(1):2178.
- Brown TL, Petrovski S, Dyson ZA, et al. The Formulation of Bacteriophage in a Semi Solid Preparation for Control of Propionibacterium acnes Growth. McDowell A, editor. PLOS ONE. 2016 Mar 10;11(3):e0151184.
- Challener CA. Balancing the Art and Science of Topical Drug Formulation. Pharm Technol. 2021 Apr;45(4):20–5.
- Carroll-Portillo A, Coffman CN, Varga MG, et al. Standard Bacteriophage Purification Procedures Cause Loss in Numbers and Activity. Viruses. 2021 Feb 20;13(2):328.
- Vandenheuvel D, Singh A, Vandersteegen K, et al. Feasibility of spray drying bacteriophages into respirable powders to combat pulmonary bacterial infections. Eur J Pharm Biopharm Off J Arbeitsgemeinschaft Für Pharm Verfahrenstechnik EV. 2013;84(3):578–82.
- Friess W, Winter G. Meeting the challenges in freeze-drying of pharmaceuticals and biologicals. Eur J Pharm Biopharm. 2013 Oct;85(2):161.
- Patel SM, Pikal MJ. Emerging Freeze-Drying Process Development and Scale-up Issues. AAPS PharmSciTech. 2011 Mar;12(1):372–8.
Related topics
Active Pharmaceutical Ingredient (API), Antibiotics, Biologics, Biopharmaceuticals, Drug Development, Formulation, Microbiology, Therapeutics