Pharmaceutical analysis with portable spectrometers
Posted: 22 February 2022 | Richard Crocombe (Crocombe Spectroscopic Consulting) | No comments yet
Richard Crocombe of Crocombe Spectroscopic Consulting surveys the use of portable spectrometers in pharmaceutical manufacturing, with an emphasis on vibrational spectroscopy. Ideal and typical features of portable spectrometers are reviewed, alongside explanation of why device characteristics and sample presentation are critical to obtaining meaningful results.
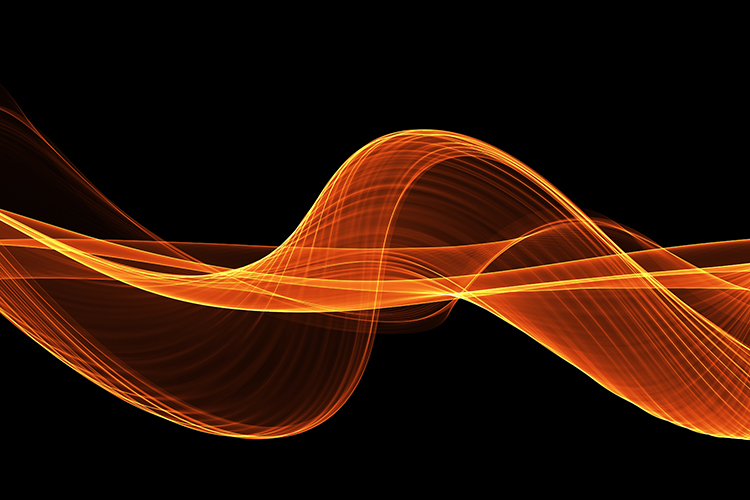
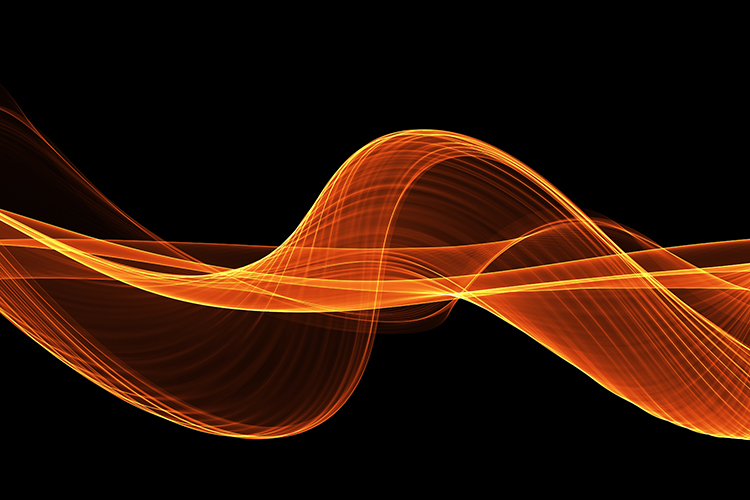
Introduction
This article describes the use of portable spectrometers in pharmaceutical manufacturing with a focus on vibrational spectroscopy: mid‑infrared (MIR), near-infrared (NIR)1,2 and Raman. Over the last twenty years, the combination of advances in consumer electronics, telecommunications optics and photonics miniaturisation has driven the development of a wide variety of portable spectrometers, from Raman, NIR and mass spectrometry to nuclear magnetic resonance (NMR) relaxometry.3 In general, the applications of portable spectrometers are diverse, from safety and security to field geology,4 but they have particular importance in the pharmaceutical industry.
Table 1 lists the ideal and possible features of portable spectrometers, which feature in many instruments currently on the market. Although hyperspectral instruments (visible, NIR, Raman) are increasingly portable and available at lower costs, these are beyond the scope of this article.
Adoption trends
Historically, running samples for MIR required a considerable amount of skilled sample handling, knowledge regarding the most suitable technique, and understanding that sample presentation is different for solids and liquids. Conversely, sample presentation for NIR instruments was very simple – merely placing the sample on the quartz window of the spectrometer. Therefore, early on, pharmaceutical QA/QC gravitated towards the use of NIR instruments5 and the spectral discrimination for QA/QC purposes was ‘good enough’, despite NIR bands being less specific and less characteristic than MIR.
Raman spectroscopy changed dramatically in the late 1980s to early 1990s with the advent of two new types of instrument: Fourier-transform Raman (FT-Raman) using 1064nm excitation; and smaller, single-stage spectrographs with the combination of charge-coupled device (CCD) detectors, efficient optical filters and 785nm solid-state lasers. These innovations started to move Raman spectroscopy from a research laboratory technique to a more routine analytical tool. The next major innovation came in the early 2000s, when fully integrated, handheld instruments (again based on 785nm excitation) were introduced. This generated competition between NIR and Raman in the chemical/pharmaceutical QA/QC area, and Raman has been increasingly used due to a combination of lower sensitivity to particle size and moisture, as well as more specific spectroscopic information (fundamentals versus overtones for Raman, and combinations in the NIR).
Compliance, conformance and qualification
Historically, running samples for MIR required a considerable amount of skilled sample handling, knowledge regarding the most suitable technique, and understanding that sample presentation is different for solids and liquids”
In the highly regulated pharmaceutical industry, it is essential to verify that an analytical instrument is fit for purpose. This encompasses many different aspects: compliance with national safety requirements and regulations (eg, CE); conformance to pharmaceutical standards (eg, European Pharmacopeia); conformance to US Food and Drug Administration (FDA) standards (21 CFR Part 11); and documentation of the product’s fitness for purpose (DQ/IQ/OQ/PQ). Here, the major vendors will supply conformance and compliance statements, as well as draft standard operating procedures (SOPs) to enable timely deployment of the instruments.
Some of the vendors of portable spectrometers enable ‘fleet management’ of deployed instruments, which includes the ability to perform regular firmware and software and library updates. For many operators of handheld spectrometers, especially those in regulated industries and law enforcement, fleet management of their instruments is essential to ensure each instrument is operating with known algorithms, libraries and related databases. This is especially important when large numbers of systems are deployed across the globe and must be feasible from a logistical perspective: in a highly regulated industry such as pharmaceutical manufacturing, or one with world-wide presence, quality supervisors must be certain that the appropriate libraries and algorithms are used.
Vibrational spectroscopy
Many reviews and books compare MIR, NIR and Raman spectroscopies from an academic or theoretical point of view, but Table 3 approaches this from a practical point of view; using portable instruments for pharmaceutical samples. The immediate conclusion is that MIR spectroscopy, while generating highly chemically specific information, is far more laborious than NIR or Raman, especially in a QA/QC environment.
For Raman spectroscopy, the largest potential problem is fluorescence,6 which can overwhelm the Raman signal. This can arise from the presence of even small amounts of coloured materials, transition metal ions, or highly conjugated or aromatic compounds. In this context it is worth noting that many pharmaceutical active ingredients (as well as common street drugs) are conjugated or aromatic molecules. Excitation at 785nm has been a good compromise between long wavelength excitation while still leveraging low-cost and noise- silicon-based detectors. Several approaches to eliminating or mitigating fluorescence are employed today, including moving to 1064nm excitation, at the expense of measurement time for comparable signal‑to‑noise spectra; deep‑ultraviolet (UV) excitation which does not work with glass containers; shifted-excitation schemes; time‑gating; and computational methods. It is not clear if there is a ‘best approach’, or what a universal solution could be. However, a potential advantage of Raman spectroscopy is its ability to interrogate a sample through translucent packaging. This is achieved via spatially offset Raman spectroscopy (SORS), which could be especially useful in incoming raw material identification.7
Raw material identification
In a production environment the return on investment (RoI) for an instrument purchase is a key consideration – a production manager will not purchase an instrument without a good ratio. For a pharmaceutical raw material identification (RMID) application, performed by handheld Raman or NIR, the benefits include ease of operation (use on loading dock, in warehouse or inspection area with ‘point-and-shoot’ sample interrogation through plastic liners); fast analysis (typically less than five seconds); accurate and reliable results (based on suppliers’ barcode, built-in library and algorithm); no waste, sample preparation or opportunity for contamination; and transferable methods. Knowledge of the current RMID procedure makes it straightforward to calculate a hypothetical RoI – this is outlined in Table 4. The assumptions here are relatively simple and this RoI is based only on operator labour and time savings. Further benefits that could improve the RoI include, but are not limited to, increasing inventory turnover; improvements in operational areas, such as warehouse space, due to the reduction in inventory volumes; and reduced routine laboratory workload, which could enable scientists to focus on problem solving instead of QA/QC.
Primary API manufacturing
The main application of vibrational spectroscopy in primary manufacturing, apart from RMID, is in reaction monitoring during the production of active pharmaceutical ingredients (APIs). This is not a portable or handheld application, but the same technologies are used in compact benchtop spectrometers, interfaced with probes mounted on reaction vessels, as those used in the laboratory, through scale‑up to production. A recent paper reviewed the role of Raman spectroscopy in the biopharmaceutical industry.8
Secondary manufacturing unit processes
In a production environment the return on investment (RoI) for an instrument purchase is a key consideration”
The use of NIR spectrometers at almost every stage of secondary (drug product) pharmaceutical manufacturing has been extensively described:9 for blending, granulation, drying, sizing, extrusion, compaction, tableting, coating, end product verification, etc. End product verification should be quantitative – and this has been demonstrated using different portable NIR spectrometers.10 Many unit operations employ process instruments, rather than portables, as noted above. The technologies that have enabled portable instruments also facilitate miniature process instruments, allowing them to be mounted on unit operations equipment which may be in motion (eg, blenders), or subject to high vibrations (eg, granulators, roller compactors and pill presses). The engineering factors – including vibration‑insensitive micro-electromechanical systems (MEMS) components or those without moving parts, which enable portable instruments to survive drops, work over variable temperatures and varying humidity, etc – also facilitate the instruments’ use in these challenging environments. Reactor clean-out verification appears to be a case for which a rapid portable solution remains wanting, although a variety of techniques, including fluorescence and ion mobility spectrometry, have been investigated.
Counterfeit detection
Field testing of suspected counterfeit pharmaceuticals is required to remove these materials from the retail chain as quickly as possible – and to deter future fraudsters.11 This is not a manufacturing application, but the instruments described above are used in the field for this screening application, as even small differences in the manufacturing process, formulation or raw materials can be detected spectroscopically. Although pharmaceutical companies do not disclose the security measures they employ to protect their supply chain, nor do government agencies and regulatory bodies publicise their methods, there are published success stories. One of the best known is the use of portable Raman spectrometers by the National Agency for Food and Drug Administration and Control (NAFDAC) in Nigeria,12 described in a Dan Rather Reports episode.13
Other spectroscopic techniques
Other portable or handheld spectroscopic techniques play a valuable role in pharmaceutical analysis, a couple of which are described herewith.
X-Ray fluorescence
X-ray fluorescence (XRF) is a mature technique for elemental analysis and portable XRF instruments have been available for more than twenty years, and widely deployed. XRF is a quantitative technique, employing both empirical and fundamental-parameter calibrations. It is a ‘point‑and-shoot’ technique, being non-invasive and non-destructive, and typically has short measurement times from a few seconds to about a minute. A limitation in the pharmaceutical industry is that its elemental range is from magnesium to uranium; XRF is insensitive to light elements, especially in a portable instrument format. XRF methodology also only indicates the presence or concentration of those specific elements and not their binding manner (ie, which anions are associated), which is important information as binding significantly impacts human uptake. XRF can also be used to check for the presence of inorganic elements in metallic packaging.
In general, solid dosage forms require some sample handling and preparation (eg, poured out from their capsule or ground up solid tablets) and the sample cup filled to a sufficient depth to give infinite thickness. In this case, quantitative analysis for elements such as Ca, K, Fe, Mg, Ti, Zn, Pt, etc, can be performed. Trace or ultra-trace elements like selenium in solid dosage forms and nutraceuticals can present a problem, due to the limit of detection or imprecision at very low concentration levels. XRF can also be used to analyse for trace metals (eg, Zn, Mn, Al and Si) in the aluminum foil in blister packs and on the caps on vials, as these trace elements will vary depending on the source of the foil or cap, which can be a counterfeit detection or confirmation measure. A product safety-related analytical test in the pharmaceutical industry is the quantification of inorganic impurities within the product. This includes toxic heavy metals, such as As, Cd, Hg, and Pb. In addition, APIs may contain residual metal catalysts, such as Ru, Pt and Pd. Since there are many potential sources of contamination, it may be of interest to measure raw materials and intermediates as well as final products.
Relaxometry
Relaxometry (or time-domain nuclear magnetic resonance: TD-NMR) is a variant of NMR, typically used to analyse moisture and fat in food samples. Relaxometers have been commercially available in compact benchtop formats for decades, and with advances in rare-earth magnet and radiofrequency (RF) technologies, this type of instrument can be miniaturised further and made portable.
TD-NMR spectroscopy has recently been suggested as a screening method to detect counterfeit biologic pharmaceuticals.14 The authors report that TD-NMR is very sensitive to differences in the amount of antibody in solution and it is therefore capable, by comparing data from known formulations, of determining whether a particular sample is likely to be of an authentic biologic formulation. This is a ‘fingerprinting measurement’ only, as TD-NMR does not provide chemical-shift information.
About the author
Richard Crocombe is the Principal at Crocombe Spectroscopic Consulting. He works with both emerging and established instrument companies on miniature optical technologies and handheld/portable spectrometers, go‑to‑market strategies and their emerging applications. Prior to this, he worked at several companies including PerkinElmer, Thermo Fisher Scientific, Axsun Technologies and Bio-Rad Laboratories, mostly concerned with development of new spectrometers and their applications. He has published extensively on the technologies and applications for miniature and portable spectrometers, including a comprehensive review article in Applied Spectroscopy in 2018. Richard Crocombe, Pauline Leary and Brooke Kammrath are the joint editors of the twovolume book, ‘Portable Spectroscopy and Spectrometry’, published by John Wiley in April 2021.
Acknowledgements
The author would like to acknowledge helpful conversations with Pauline Leary (Federal Resources), Debbie Schatzlein Griggs (Rigaku) and Michael Gallagher (Thermo Fisher Scientific).
References
- Beć K, Grabska J, Huck C. (2020). Principles and Applications of Miniaturized Near‐Infrared (NIR) Spectrometers. Eur. J.2021; 27: 1514-1532. Available from: doi: 10.1002/chem.202002838
- Beć K, Grabska J, Siesler HW, Huck CW. Handheld near-infrared spectrometers: Where are we heading? NIR News. 2020; 31(3-4):28-35. Available from: doi:1177/0960336020916815
- Crocombe RA, Leary PE, Kammrath BW, Editors. Portable Spectroscopy and Spectrometry, Volume 1, Technologies and Instrumentation. Chichester UK and Hoboken NJ: John Wiley; 2021. ISBN: 978-1-119-63641-0.
- Crocombe RA, Leary PE, Kammrath BW, Editors. Portable Spectroscopy and Spectrometry, Volume 2, Applications, Chichester UK and Hoboken NJ: John Wiley; 2021. ISBN: 978-1-119-63642-7.
- Ciurczak EW, Igne B. Pharmaceutical and Medical Applications of Near-Infrared-Spectroscopy, Second Edition, Boca Raton: CRC Press (Taylor and Francis Group), 2015. ISBN 9780367377977
- Rathmell C, Bingemann D, Creasey D. Portable Raman Spectroscopy: Instrumentation and Technology, Chapter 6 in Crocombe RA, Leary PE, Kammrath BW, Editors. Portable Spectroscopy and Spectrometry, Volume 1, Technologies and Instrumentation. Chichester UK and Hoboken NJ: John Wiley; 2021. ISBN: 978-1-119-63641-0.
- Hargreaves M. Handheld Raman, SERS and SORS, Chapter 16 in Crocombe RA, Leary PE, Kammrath BW, Editors. Portable Spectroscopy and Spectrometry, Volume 2, Applications, Chichester UK and Hoboken NJ: John Wiley; 2021. ISBN: 978-1-119-63642-7.
- Esmonde‑White KA, Cuellar M, Lewis IR. The role of Raman spectroscopy in biopharmaceuticals from development to manufacturing, Anal Bioanal Chem.2021; Available at: https://org/10.1007/s00216-021-03727-4
- Sacre PY, De Bleye C, Hubert P, Ziemons E. PAT Applications of NIR Spectroscopy in the Pharmaceutical Industry, Chapter 4 in in Crocombe RA, Leary PE, Kammrath BW, Editors. Portable Spectroscopy and Spectrometry, Volume 1, Technologies and Instrumentation. Chichester UK and Hoboken NJ: John Wiley; 2021. ISBN: 978-1-119-63641-0.
- Yan H, Siesler HW. Quantitative analysis of a pharmaceutical formulation: Performance comparison of different handheld near-infrared spectrometers, Journal of Pharmaceutical and Biomedical Analysis.2018: 160: 179-186. Available at: https://doi.org/10.1016/j.jpba.2018.07.048.
- Leary PE, Crocombe RA, Kalyanaraman R. The Value of Portable Spectrometers for the Analysis of Counterfeit Pharmaceuticals, Chapter 5 in Crocombe RA, Leary PE, Kammrath BW, Editors. Portable Spectroscopy and Spectrometry, Volume 2, Applications, Chichester UK and Hoboken NJ: John Wiley; 2021. ISBN: 978-1-119-63642-7.
- Bate R. PHAKE: The Deadly World of Falsified and Substandard Medicines. Washington, D.C.: The AEI Press; 2014.
- Rather D. (2010, September 14). The Mysterious Case of Kevin Xu. Dan Rather Reports. AXS TV. This section begins at about six minutes into the report. Available at: https://www.youtube.com/watch?v=JL9iiyAx3Qw
- Akhunzada Z, Wu Y, Haby T, et al. (2021). Analysis of Biopharmaceutical Formulations by Time Domain Nuclear Magnetic Resonance (TD-NMR) Spectroscopy: A Potential Method for Detection of Counterfeit Biologic Pharmaceuticals. J Pharm Sci. 2021; 110(7): 2765-2770. Available at: doi: 10.1016/j.xphs.2021.03.011.
Issue
Related topics
Active Pharmaceutical Ingredient (API), Analytical techniques, Drug Manufacturing, Drug Safety, Lab Equipment, Near Infrared Spectroscopy (NIR), QA/QC, Raman Spectroscopy, Spectroscopy, Technology, Therapeutics
Related organisations
European Pharmacopoeia (Ph. Eur.), US Food and Drug Administration (FDA)