Challenges in downstream purification of advanced therapies
Posted: 22 February 2022 | John Liddell (CPI) | No comments yet
John Liddell, Chief Technologist at the Centre for Process Innovation (CPI), writes about the challenges associated with gene therapy products, which constitute a major portion of the overall cell and gene therapy market.
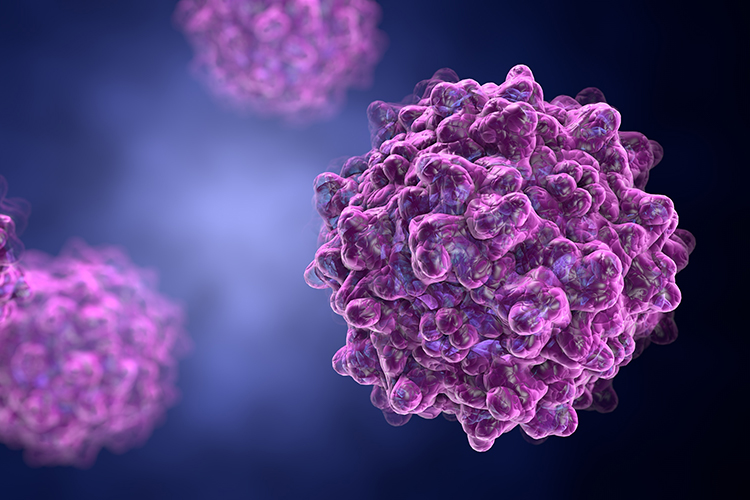
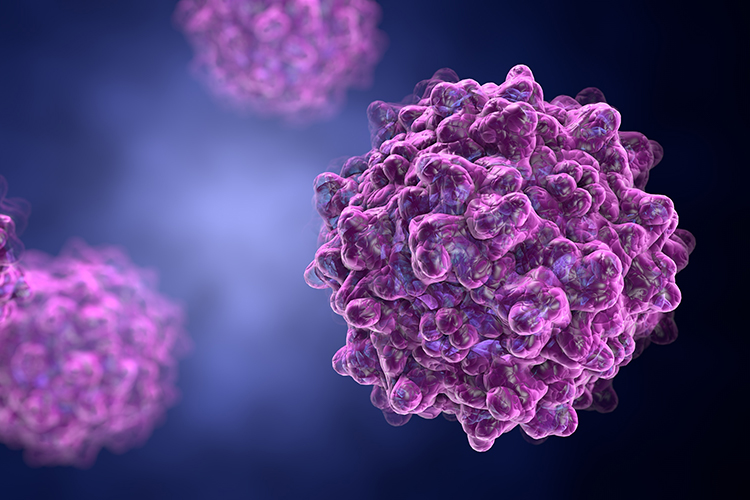
CELL AND GENE therapy products are innovative and complex biological products that aim to revolutionise medicine, meeting unmet medical needs as well as providing curative treatments. The area has attracted significant interest and is growing at a compound annual growth rate (CAGR) of 54 percent and estimated to be valued at $59 billion by 20261 (Figure 1). However, the complexity of these revolutionary products presents manufacturing challenges, especially for downstream processing.
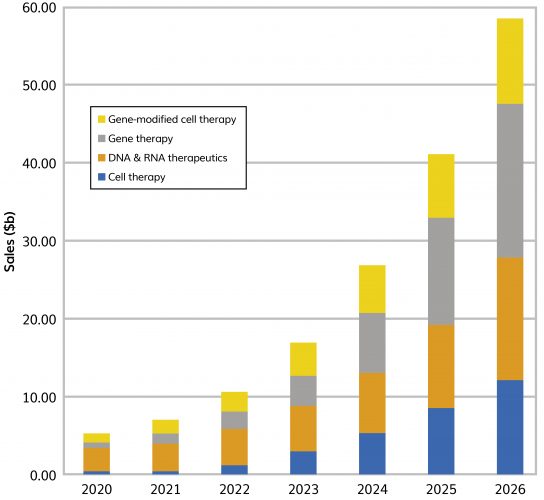
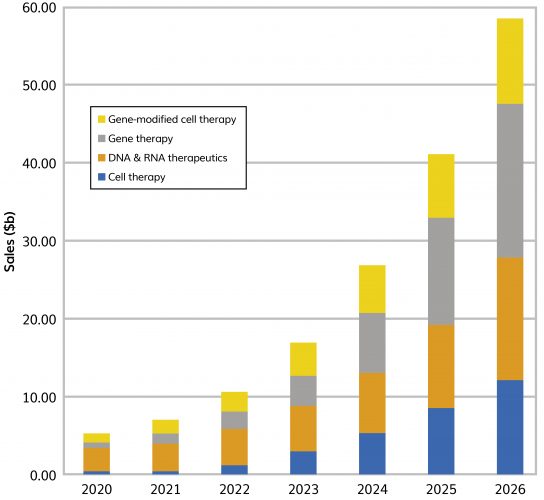
Figure 1: Current and predicted sales of cell, gene, regenerative and nucleic acid therapy products (2020–2026). Source: EvaluatePharma, July 2021
Gene therapy products
Some examples of licensed gene therapy products2 are shown in Table 1.
As shown in the table, viral vectors are the preferred route to deliver gene therapy products to the patient, with the three most common types of gene therapy vectors being adenovirus, adeno‑associated virus (AAV) and lentivirus (LV). Some basic parameters of these viruses are summarised in Table 2.
The following two basic approaches for viral gene therapy are used:
- In vivo gene therapy. A gene is introduced into the patient using a viral vector to infect cells and deliver the gene. This is typically to increase or replace low levels of the protein encoded by the transgene. Luxturna and Zolgensma are examples of in vivo gene therapy.
- Ex vivo gene therapy. Cells are abstracted from the patient and treated with a viral vector, which delivers the gene to the abstracted cells. These modified cells are then returned to the patient. Chimeric antigen receptor (CAR)-T therapy is the main example of this, where an LV is used to deliver a gene to patients’ T cells, with Kymriah being an example.
With protein biomolecules such as antibodies, molecular structures are assessed at the amino acid level and exact binding locations can be determined for chromatography resins. Viral vectors provide a further level of complexity, being nanoparticles composed of tens to hundreds of individual proteins that are arranged in unique three-dimensional structures.
The downstream challenges for all the viral gene therapies are similar, differing in details depending on the type of viral vector being used, which is subject to the gene being delivered and the therapy type (in vivo/ex vivo). The challenges all relate to the size and complexity of the viral vector compared to a purely protein product.
Downstream purification challenges
Many current therapies target ultra‑rare diseases, treating small patient populations with small amounts of viral vector. Traditionally, purification strategies have been focused on viral size and density properties involving the use of density gradient ultracentrifugation, a lab-scale technique which must be scaled out rather than scaled up to increase throughput. Figure 2 shows a typical current process for AAV purification. Ultracentrifugation will separate empty capsids (inactive) from transgene‑containing capsids (active), making use of the small density difference between empty capsids (1.32 g/cm3) and full capsids (1.40 g/cm3). The step is slow (several hours) and requires several rounds to achieve the required capsid resolution. Chemicals used to form gradients such as caesium chloride are expensive and need to be removed from the final product. Processing is manually intensive and difficult to automate.
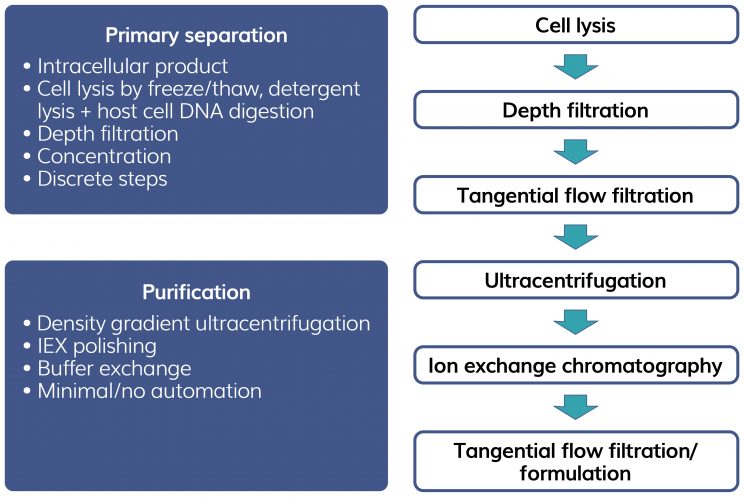
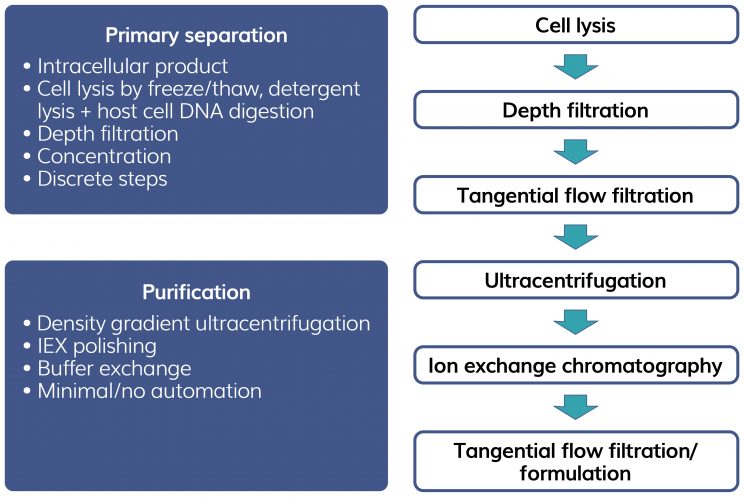
Figure 2: Example of current AAV purification processes.
All this contributes to the high cost of gene therapy products (Zolgensma therapy costs $2.1m) making them suitable only for conditions that require small doses of viral vector. Treating larger indication monogenetic diseases such as haemophilia, Duchene’s or Parkinson’s disease requires more scalable purification methods.
Alternative approaches for downstream processing of viral vectors to address this have been built using knowledge gained from recombinant protein downstream purification, which are based on multi-stage chromatography.
Achieving clearance of host cell components (proteins, nucleic acids) and control of species related to the required product are all necessary, together with additional requirements for resolution of empty capsids from transgene-containing capsids.
Below, I discuss approaches to simplify viral vector purification and achieve cost‑effective scale up.
Viral vector chromatography
Chromatography matrices for viral vectors
Protein purification has typically used chromatography media in the form of porous particles with convective flow through the beads, which enables the full bead surface area to be accessible for adsorption. Protein purification bead pore sizes are 15-40nm, meaning that viral vectors’ adsorption will be restricted to the bead surface, limiting capacity. While pore size can be increased, this reduces the structural integrity of the beads making them difficult to pack into chromatography columns. Alternative chromatography stationary phase formats based on membranes,3 monoliths4 or fibres5 have also been demonstrated with viral vectors. All can be derivatised with the typical ligands used in bead‑based chromatography – ion exchange, hydrophobic interaction, mixed mode, etc. These non-beaded formats do not suffer from the issues of pore diffusion to promote adsorption and are scalable.
Affinity chromatography for viral vectors
Affinity chromatography based on protein A binding to the Fc region of human IgG molecules is a highly effective capture step applicable to all monoclonal antibody (mAb) products, resulting in a product that is >98 percent pure. This platform process decouples all development and manufacturing of monoclonal antibodies from a specific molecule.
This platform type approach is very attractive for viral vectors. Good progress has been made in developing first-generation affinity ligands for AAV, which are available commercially. These use domain antibody fragments that have been identified to bind to AAV capsid proteins. Affinity ligands have been developed that will bind specific AAV serotypes (8,9) or groups of serotypes (1-5, 1-9). AAV is bound at neutral pH and eluted by reducing the pH to 2-3.6 Careful control of product elution is necessary to minimise the time that AAV is at low pH, which can cause deactivation. The existence of multiple serotypes means that at present a true affinity platform approach can only be applied with a need to optimise conditions of binding and elution for individual cases. Currently all these are attached to beaded chromatography matrices, which come with the disadvantages discussed above.
Empty/full capsid resolution
As well as a density difference between full and empty capsids, there is also a charge difference with the isoelectric point (pI) for empty capsids being 0.4 pH units different from full capsids. This can be used in defining a separation approach based on ion exchange chromatography. The preferred chromatography mode is anion exchange operated at a pH around 9, where in a long shallow gradient empty capsids elute earlier than full capsids. The length of the gradient required (100CV) is impractical for manufacturing but having identified the conditions for elution of empty and full capsids, subsequent conversion to a step gradient gives a scalable approach to capsid resolution.7
Viral vector size
The size of viral vectors compared to other species that come from the host cell, such as proteins and post nuclease digestion nucleic acid fragments, means that tangential flow filtration can achieve significant viral vector purification from host cell impurities. Having conditions that avoid viral vector aggregation while still reducing binding of host cell components to the viral vector will involve innovative buffer selection.
Future prospects
Having demonstrated the clinical efficacy that viral vectors can bring to unmet medical need, the downstream challenge is to achieve lower manufacturing costs with scalable processes that deliver products of the high quality required of a therapeutic. As seen above, the components are all present but require further refinement and development.
The size of viral vectors compared to other species that come from the host cell, such as proteins and post nuclease digestion nucleic acid fragments, means that tangential flow filtration can achieve significant viral vector purification from host cell impurities”
Affinity chromatography has been demonstrated but may be most efficiently used with non-beaded supports. Empty/full separation by chromatography is achievable as outlined above but will likely require case-dependent development. This aspect of serotype‑dependent behaviour presents one of the main challenges for AAV. Since clinical applications will require different serotypes to be used to target different tissues, the development of serotype-independent robust platforms for purification that are suitable for varieties of AAV serotypes remains a development goal.
The current state of affinity chromatography technology does not differentiate between full and empty capsids. Here, the development of resins with selectivity for full capsids would be a significant breakthrough to streamline viral vector downstream workflows.
New modalities in gene therapy
This brief review has focused on viral vectors and specifically AAV for gene therapy delivery, but the area of gene delivery is very dynamic and two areas have been attracting significant attention.
The first is the use of extracellular vesicles (exosomes) as a useful vector for therapies because they are composed of cell membranes and are well tolerated by the host. The cellular membranes have multiple adhesive proteins on their surface and provide an exclusive approach for targeted delivery. Additionally, they can cross the blood brain barrier, which is not currently possible with other delivery approaches. The purification challenge for exosomes as potential therapies is the separation from other extracellular vesicles – an area where affinity chromatography targeting exosome surface markers would be a significant development.
The second area of attention is messenger RNA (mRNA) where focus has been on vaccine applications for disease. Application in pandemic vaccines has accelerated the development of mRNA therapies and delivery by lipid nanoparticles (LNP), and focus on the technology is likely to expedite applications in cancer immunotherapies, therapeutic protein replacement therapies and the treatment of genetic diseases.
mRNA downstream challenges again relate to the size of the molecule, which would be around 40-60nm in size and with molecular weights of 4-5MDa. The manufacturing process is significantly different from viral vectors, being an enzymic‑based synthesis from a DNA template. Again, applications for affinity chromatography have proved useful with the use of poly thymidine bound to chromatography media being used to purify mRNA through the poly adenosine tail, which must be present for mRNA to be used effectively.8
Conclusions
The clinical interest in cell and gene therapies is evident by the fact there are more than 1,000 therapies in the development pipeline. However, to achieve their promise of providing durable curative treatments requires attention to scalable manufacture to address current issues of cost and availability. Improvements to downstream purification as outlined in this article will make a major contribution to achieving this.
About the author
John Liddell is Chief Technologist at the Centre for Process Innovation (CPI), a UK-based technology innovation centre and part of the UK Catapult network. He has experience of a wide range of biopharmaceuticals, including novel protein formats, antibodies, viral vectors, vaccines and mRNA technology. He provides support to biopharmaceutical development to increase access to new therapies through the application of innovative technologies. John’s previous experience involved the development and manufacturing of biologics, working in senior process development roles with global organisations, including Fujifilm Diosynth Biotechnologies, Merck & Co, Avecia Biologics, Zeneca and ICI. He has almost 30 years of experience in bioprocessing with direct involvement in all phases of bioprocess development from early-phase process definition to late-phase process validation and commercial production.
References
- Verdin P, Mon Tsang T. “Next-generation therapeutics thrust into the spotlight” Biopharma Dealmakers. 2021.
- American Society of Cell and Gene Therapy ASCGT Gene, Cell, & RNA Therapy Landscape Q3 2021 Quarterly Data Report
- Douglas, et al. Vaccine 37 (2019) 6951–6961 “Simian adenovirus vector production for early-phase clinical trials: A simple method applicable to multiple serotypes and using entirely disposable product-contact components”
- Gagnon P, et al. “Streamlining Industrial Purification of Adeno-Associated Virus” BioProcess Int Dec 17 (2020)
- Turnbull, et al. “Adenovirus 5 recovery using nanofiber ion-exchange adsorbents” Biotechnol Bioeng. (2019) 116 1698-1709
- McKenna, et al. Molecular Therapy: Methods & Clinical Development 19 (2020) “Characterization of AAV-Specific Affinity Ligands: Consequences for Vector Purification and Development Strategies”
- Bakhshayeshi, et al. Biotech J 16 (2021) “Separating Empty and Full Recombinant Adeno-Associated Virus Particles Using Isocratic Anion Exchange Chromatography”
- Flook K. “Supporting development of mRNA-based therapies by addressing large-scale purification challenges” Cell & Gene Therapy Insights 2021; 7(5), 489–502
Issue
Related topics
Biologics, Biopharmaceuticals, Bioprocessing, Bioproduction, Chromatography, Drug Manufacturing, Gene therapy, Industry Insight, Lab Equipment, Regenerative Medicine, Technology, Therapeutics, Viruses
Related drugs
Related diseases & conditions
Cancer, Duchenne muscular dystrophy (DMD), Genetic disorders, haemophilia, Parkinson's disease