Pharmaceutical salts of small molecule drugs: opportunities and challenges
Posted: 28 October 2014 | | No comments yet
Over the past decades, pharmaceutical drug development has undergone some significant changes, a prominent example for this being the emergence of biomolecular drugs (New Biological Entities, NBEs) such as antibodies and peptides. However, classical small molecule drugs (New Chemical Entities, NCEs) are far from being a dying species, and in fact the number of NCE drugs approved by the US Food and Drug Administration (FDA) in the past few years has been higher with increasing trend compared to NBE drugs, which appear to stagnate at lower level in terms of new drug approvals1.
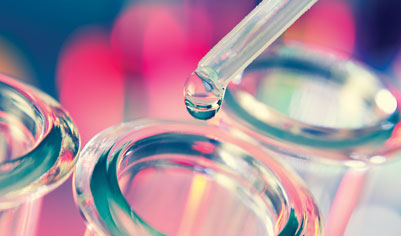
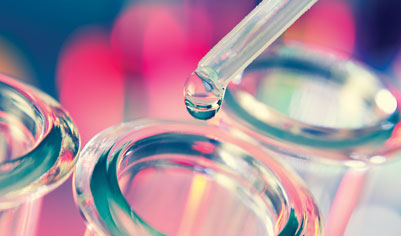
For NCE drugs, however, the introduction of High-Throughput (HT) combinatorial chemistry platforms as well as HT assay technologies has resulted in a pronounced increase in poorly water-soluble drugs, e.g. BCS class 2 and class 4 compounds2,3,4.
Hence, pharmaceutical development is nowadays frequently facing the challenge of tailoring poorly soluble lipophilic drugs to allow sufficiently high oral in-vivo absorption. This is even more demanding for early animal toxicological studies which typically require high doses being absorbed. Enabling formulation platforms are widely considered to mitigate this, and formulation scientists have a diverse tool-box available in this field, such as liquid formulation approaches, amorphous molecular dispersions, or particle size reduction technologies5,6. However, nearly all of these enabling formulation platforms require considerable development time and workload, which may be prohibitive especially for early-stage development timelines. Also, some of these approaches are limited in the applicability (e.g. particle size reduction is only useful if dissolution kinetics are the main limitation for oral absorption, but does not overcome solubility constraints itself). Moreover, one of the main liabilities of many enabling formulation products is the risk with respect to stability (physical form conversion issues in case of amorphous solid drug dispersions, enhanced chemical reactivity in solution state).
Alternatively, going one step back from formulation development activities, modification of drug substance (DS) solid-state properties may be considered as a more direct tool to overcome poor solubility. Although in principle this comprises a multitude of possible solid-state forms as well7, typically small solubility differences will be observed between polymorphic forms or anhydrous versus hydrate forms8. Hence, for overcoming solubility limitations from a DS solid-state perspective, amorphous phase and salt formation are the two most effective options. Whereas the former will inevitably raise stability concerns upon processing and storage (mainly with respect to risk of crystallisation), the latter is widely utilised. In fact, approximately half of all drugs listed in the FDA Orange Book were shown to represent salt entities, with hydrochloride salt forms being the overwhelming dominant salt entity for API bases and sodium salts being the dominant salt entity for API acids9.
For all these approaches, NCE drug development is subject to increasing regulatory requirements. Most notably the quality by design (QbD) concept is strongly encouraged in ICH Q8(R2) guideline to demonstrate a sound scientific understanding of all relevant steps of drug development10. In this context, it is also evident that a deeper understanding of solid-state selection and properties of solid-state forms is required.
Some of the main opportunities and challenges associated with a systematic salt selection process will be outlined in more detail.
Improving dissolution behaviour
Pharmaceutical salt selection is extensively described in literature for overcoming poor solubility11,12. In this context, sulfonate salts are advocated as especially useful counter ions for basic drugs13. However, at a given pH and counter ion milieu, thermodynamic solubility of an API entity should be the same whether it is obtained for a neutral species or a corresponding salt entity, as this merely refers to the equilibrium solubility between solution state and whichever solid-state form is thermodynamically stable in the given milieu. In fact, what is really impacted by salt formation is the dissolution rate of the solid-state phase, which is typically higher in salt entities compared to neutral species for three main reasons: (a) ionic interactions in the salt crystal lattice increases hydrophilicity in the powder and can thus facilitate wettability, (b) presence of dissolved counter ion in the diffusion layer shifts the micro-environmental pH milieu towards higher solubility pH domains for the drug, (c) significant hydration energy of polar counter ions typically contribute to the overall net gain in Free Energy levels upon dissolution process.
As enhanced dissolution rates often result in intermediate supersaturation levels from a thermodynamic point of view, this poses the imminent risk of precipitation of the free form. This is especially the case for dissolution / solubility processes of salt entities of rather weak drug bases (with basic pKa values <7, which accommodates for a main fraction of modern API drugs) in intestinal aqueous media (e.g. Simulated Intestinal Fluid [SIF], pH 6.8), where from thermodynamic perspective the free form should govern the equilibrium solubility. Hence, the extent to which poor solubility is effectively addressed will depend on the tendency of a certain salt entity to withstand precipitation processes. This, however, is often not so much of an issue under in-vivo-like conditions, since the presence of natural surfactants such as sodium taurocholate and lecithine may act as solubilisers and precipitation inhibitors. This is demonstrated in Figure 1 by comparison of in-vitro dissolution profiles for a weakly basic API (pKa value ~6) and its phosphate salt in Simulated Intestinal Fluid (SIF, pH 6.8) versus Fasted-state Simulated Intestinal Fluid (FaSSIF, pH 6.5), the latter mimicking more closely in-vivo milieu14.
Solid-state properties (crystallinity, polymorphism, particle morphology)
Salt formation can be an effective tool to obtain higher crystalline solid-state forms. This can be illustrated by a merely theoretical consideration: introduction of ionic interactions into the crystal lattice of salt entities may be considered beneficial in terms of crystal lattice stabilisation, as permanent ion-ion interaction will contribute stronger to lattice enthalpy than non-ionic interactions such as hydrogen bonding, p-stacking, or van-der-Waals interactions. In fact, it is sometimes observed during salt crystallisation processes that spontaneous crystallisation sets in when adding counter ion solutions to solutions of free forms in organic media, as the stronger lattice interaction in the salt crystals is the driving force for the poorer solubility behaviour in organic media. However, obtaining a highly crystalline salt form depends to large extent on finding the right crystallisation conditions as well.
From a theoretical perspective, formation of the most favourable ionic lattice interactions in salt crystal structures should also strongly determine the preferred packing arrangement of a given drug / counter ion / stoichiometry combination, and hence one may further expect a lower tendency of salt entities to exhibit polymorphism. Statistical analysis of non-salt drugs vs salt drugs listed in the European Pharmacopoeia (edition 4.02) seems to support this trend15. However, it must be noted that polymorphism is a result of several complex interactions, e.g. lattice contributions through favourable hydrogen bonding interaction patterns as well as the dualism of lattice enthalpy vs entropy stabilization, and there are also numerous examples for polymorphic behaviour in salt forms of prominent marketed drugs (e.g. Clopidogrel hydrogensulfate, Ranitidine hydrochloride, Enalapril maleate, Atorvastatin calcium).
Moreover, in the context of solid-state form control, salt formation often bears the liability of enhanced tendency for hydrate formation15. The reason for high affinity of salt structures to incorporate water into their crystal lattice is a consequence of a strong interaction potential of water with ions in the salt structure, especially with metal cations in acidic drug salts16. Additionally, a second aspect must be considered: Many drug structures typically bear an imbalance between a larger number of hydrogen bond acceptors and lower number of hydrogen bond donors (e.g. hydroxyl protons, amino protons). During salt formation, introduction of highly polar counter ions with typically large numbers of lone electron pairs further increases the need for hydrogen bond donors to realise optimal coordination numbers in the counter ions, which often triggers incorporation of water or polar solvent molecules with additional hydrogen bond donor potential. Hence, for salt optimisation, it is essential to study any salt forms with respect to hydrate and solvate formation tendency. According to the author’s experience, these factors are the main rule-out criterion for salt forms from a quality perspective (besides lack of physical stability which is addressed below).
Salt formation can offer opportunities to overcome non-desirable particle properties of free form drugs, which may limit the API manufacturability during drug product processing. One example for this is potential for triboelectric charging, which is frequently observed in lipophilic free form solids. In contrast to this, as salt forms tend to adsorb higher levels of water on the particle surfaces, electrostatic discharging upon mechanical processing is effectively facilitated in salt entities17. Also, certain particle shapes may be problematic for manufacturing, most notably needles and rods which are especially unpopular with formulation scientists because of poor powder flowability. Although particle morphology will not merely depend on the solid-state form as such, but also on the crystallisation conditions utilized (e.g. nature of solvent, level of supersaturation), some forms can exhibit a high persistence to crystallise in highly specific morphologies such as needles. Figure 2 illustrates an impressive example where salt formation was key to overcome the needle-like particle morphology of the free form, which was consistently observed for this drug regardless of crystallisation process utilised.
Stability aspects (chemical, physical)
As salt formation typically strongly alters the solid-state interactions, it appears plausible that stability behaviour of salt forms may be somewhat different to the corresponding free form. However, it is by no means clear-cut that salt formation will inevitably yield forms with increased stability compared to their free form parents, as it will be outlined in the following section.
On the one hand, salt formation can be a major contribution to stabilise an API solid-state form towards chemical degradation processes. An impressive example for this is the world’s best-selling drug from recent years, Atorvastatin, where salt formation as calcium or sodium salt is strongly contributing to avoid lactone formation, which is a commonly observed degradation pathway in most statins under acidic conditions. However, on the other hand, introduction of counter ions in close proximity to typically rather reactive functional groups of the API (which may account for most basic or acidic functional groups utilised for salt formation) can also offer completely new degradation pathways due to covalent bond formation between counter ion and drug (e.g. amide formation).
The far more relevant liability of salt forms nowadays with respect to stability behaviour is potentially insufficient physical stability of salt forms towards dissociation to free form entity18. This is even more prevalent as the majority of today’s drugs can be classified as weakly alkaline or weakly acidic drugs (weakly being defined as corresponding pKa values ranging from 4.5 – 9.5)19. Although pKa value is somewhat indicative for the likelihood to encounter physically stable salt forms, it is pHmax which accurately describes the threshold pH level below which (for basic drugs) or above which (for acidic drugs) a salt form is thermodynamically stable towards hydrolysis12. In this concept, it is important to realise that for a given API structure, different salt entities (i.e. different counter ions) will exhibit different pHmax threshold levels, and it is not necessarily desirable to opt for the salt entity with highest solubility improvement, as this will inevitably narrow the pH-window of thermodynamic stability for such a salt entity (see Figure 3).
For many weakly basic or weakly acidic API structures for which salt formation was pursued, salt hydrolysis to free form entity is a latent risk especially during formulation process, if for instance a wet granulation process is utilised or alkaline excipients are involved in the formulation (e.g. magnesium stearate as lubricant). The importance to understand such conversion behaviour is highlighted by the FDA perspective during MAA registration of the platelet inhibitor Prasugrel in 200920. For this product, the initially formulated HCl salt exhibited high degree of conversion to free base in the formulation upon stability investigations, prompting the FDA to request a specification limit of free base formation in the drug product as post-marketing commitment. Hence, monitoring of potential API salt form conversion to free base is highly recommendable with suitable solid-state selective tools (for instance Powder X-Ray Diffraction, Raman spectroscopy, solid-state NMR spectroscopy) to gain an understanding of salt form stability behaviour in final dosage forms. At the same time, it is of paramount importance to carefully select appropriate formulation conditions (e.g. type of excipients, formulation process) as well as suitable container closure system (e.g. protective moisture barrier, use of desiccant) to reduce potential risk of salt hydrolysis in formulations.
Co-crystals as alternatives to salt forms?
Pharmaceutical co-crystals as mixed crystal systems between drugs and non-active co-formers via non-ionic interactions have gained a lot of attention in recent years in solid-state research activities21. Indeed the perspective of tailoring solid-state forms through interaction with non-active neutral molecules appears to significantly broaden the list of co-formers being available for such an approach compared to the relatively limited list of pharmaceutically accepted acids and based in salt formation.
The second, probably more relevant aspect of this trend is the fact that the co-crystal approach does not exclude any neutral compound from solid-state selection processes via co-formers, as was traditionally employed in salt selection where compounds with very weak acidic or basic moieties were considered as being non-ionisable for salt formation. This paradigm is not exactly true anymore, as the potential for co-crystal formation can be seen as a viable solid-state optimisation route for practically all compounds.
Where there is light, there are shadows as well, and the shadows of pharmaceutical co-crystals are mainly twofold:
(a) As crystal lattice interactions in co-crystal formation are of non-ionic nature, the enthalpic driving force for such co-crystal systems to form a mixed crystal system is pronouncedly less than in salts. This makes co-crystal preparation a much more tedious approach with respect to finding the right crystallisation conditions, which is also a heavy challenge for finding robust up-scale conditions.
(b) There is a lot of uncertainty as to how to address co-crystals regulatory-wise, since to the author’s knowledge, there is no pharmaceutical co-crystal registered as yet for market authorisation. The recently published FDA industry guidance on their regulatory classification of co-crystals has probably further manifested this uncertainty, as the FDA takes a completely different view-point on co-crystals than on salts. In this guidance, co-crystals are merely being considered as drug-product intermediates, i.e. as a formulation product rather than a drug substance modification approach22. This has sparked a vivid discussion in the pharmaceutical solid-state community23, and the extent of future progress on this topic remains to be seen.
Finally, in this context, it should be noted that regulatory discussions may also arise from the selection of certain salt counter ions, as the acceptance of some counter ions is under scrutiny for different kind of reasons (e.g. oxalate due to potential risk of kidney failure). Hence, aspects of potential counter ion specific side effects have to be considered24. Which counter ions and co-formers to use will also strongly depend on parameters such as indication area and anticipated dose level. As a starting point for such considerations, a recent review compiled counter ion specific maximum daily intake levels from registered salt entities in the US Orange Book25.
Conclusion
Although pharmaceutical salt selection is a widely established tool for drug development of small molecule drugs, this approach is by no means as simple and straightforward as one might assume. Although improvement of dissolution and solubility behaviour is frequently achieved, other critical quality attributes such as stability or hygroscopicity may be jeopardised, which makes salt selection a rather multi-dimensional approach. Progresses in pharmaceutical co-crystal engineering have further broadened the solid-state selection tool box, however, this approach brings its own liabilities such as challenging crystallisation process development and high uncertainty with respect to regulatory classification.
References
- Mullard, “2012 FDA drug approvals”, Nature Review Drug Discovery 2013, 2, 87-91
- A. Lipinski, F. Lombardo, B.W. Dominy, P.J. Freeney, “Experimental and computational approaches to estimate solubility and permeability in drug discovery and development setting”, Advanced Drug Delivery Reviews 1997, 23, 3-25
- A. Lipinski, “Drug-like properties and the causes of poor solubility and poor permeability”, Journal of Pharmacological and Toxicological Methods 2000, 44, 235-249
- I. Oprea, “Current trends in lead discovery: Are we looking for the appropriate properties?”, Journal of Computer-Aided Molecular Design 2002, 16, 325-334
- Kawabata, K. Wada, M. Nakatani, S. Yamada, S. Onoue, “Formulation design for poorly water-soluble drugs based on biopharmaceutics classification system: Basic approaches and practical applications”, International Journal of Pharmaceutics 2011, 420, 1-10
- D. Dai, C. Pollock-Dove, L.C. Dong, S. Li, “Advanced screening assays to rapidly identify solubility-enhancing formulations: High-throughput, miniaturization and automation”, Advanced Drug Delivery Reviews 2008, 60, 657-672
- Rodríguez-Spong, C.P. Price, A. Jayasankar, A.J. Matzgar, Naír Rodríguez-Hornedo, “General principles of pharmaceutical solid polymorphism: a supramolecular perspective”, Advanced Drug Delivery Reviews 2004, 56, 241-274
- Pudipeddi, A.T.M. Serajuddin, “Trends in solubility of polymorphs”, Journal of Pharmaceutical Science 2005, 94, 929-939
- S. Paulekuhn, J.B. Dressman, C. Saal, “Trends in active pharmaceutical ingredient salt selection based on analysis of the Orange Book database”, Journal of Medicinal Chemistry 2007, 50, 6665-6672
- ICH Harmonised Tripartite Guideline, “Pharmaceutical Development Q8(R2),current step 4 version dated August 2009”, 2009 http://www.ich.org/products/guidelines/quality/article/quality-guidelines.html
- P. Elder, R. Holm, H. Lopez de Diego, “Use of pharmaceutical salts and cocrystals to address the issue of poor solubility”, International Journal of Pharmaceutics 2013, 50, 6665-6672
- T.M. Serajuddin, “Salt formation to improve solubility”, Advanced Drug Delivery Reviews 2007, 59, 603-616
- P. Elder, E. Delaney, A. Teasdale, S.Eyley, V.D. Reif, K. Jacq, K.L. Facchine, R. Schulte Oestrich, P. Sandra, F. David, “The utility of sulfonate salts in drug development”, Journal of Pharmaceutical Sciences 2010, 99, 2948-2961
- Jantratid, N. Janssen, C. Reppas, J.B. Dressman “Dissolution media simulating conditions in the proximal human gastrointestinal tract: an update”, Pharmaceutical Research 2008, 25, 1663-1676
- J. Griesser “The importance of solvates”, in: R. Hilfiker (ed.) “Polymorphism in the Pharmaceutical Industry”, Wiley-VCH, 2006
- R. Morris“Structural aspects of hydrates and solvates”, in: H.G. Brittain (ed.) “Polymorphism in Pharmaceutical Solids”, Marcel Dekker Inc., 1999
- Karner, N.A. Urbanetz, “The impact of electrostatic charge in pharmaceutical powders with specific focus on inhalation-powders”, Journal of Aerosol Science 2011, 42, 428-445
- A. Stephenson, A. Aburub, T.A. Woods, “Physical stability of salts if weak bases in the solid state”, Journal of Pharmaceutical Sciences 2011, 100, 1607-1617
- B. Maurin, D.J.W. Grant, P.H. Stahl, “The physicochemical background: fundamentals of ionic equilibria”, in: P.H. Stahl, C.G. Wermuth (eds.) “Handbook of Pharmaceutical salts”, Wiley-VCH., 2002
- FDA Center for Drug Evaluation and Research, “Application Number: 22-307, Summary Review”, 2009
- Schultheiss, A. Nwman, “Pharmaceutical cocrystals and their physicochemical properties”, Crystal Growth and Design 2009, 9, 2950-2967
- FDA Center for Drug Evaluation and Research, “Guidance for industry: Regulatory classification of pharmaceutical co-crystals (April 2013)”, 2013 http://www.fda.gov/downloads/Drugs/Guidances/UCM281764.pdf
- J. Zaworotko et al, “Polymorphs, Salts, and Cocrystals: What’s in a Name?”, Crystal Growth & Design 2012, 12, 2147-2152
- A. Thackaberry, “Non-clinical toxicological considerations for pharmaceutical salt selection”, Expert Opinion Drug Metabolism Toxicology 2012, 8, 1419-1433
- Saal, A. Becker, “Pharmaceutical salts: A summary on doses of salt formers from the Orange Book”, European Journal of Pharmaceutical Sciences 2013, 49, 614-623
Biography
Axel Becker obtained his degree in Analytical Chemistry at Reutlingen University of Applied Sciences in 2000. After spending 15 months at Henkel KGaA, working in Technical Research on colloid and interface problems, he joined Merck KGaA in 2001. At Central Analytical Services he is strongly involved in solid-state selection and physical properties characterisation of research compounds and development candidates. From 2005 to 2007, Axel spent a two-year secondment at Merck Generics UK, working on physical properties characterisations and Intellectual Property aspects of generic drugs.