Meeting biopharmaceutical analytical requirements for subvisible particle sizing and counting
Posted: 22 October 2015 |
Quantifying and sizing subvisible particles in biopharmaceutical products are crucial aspects of formulation development, stability studies, process development, product release and extended characterisation of the final drug product.
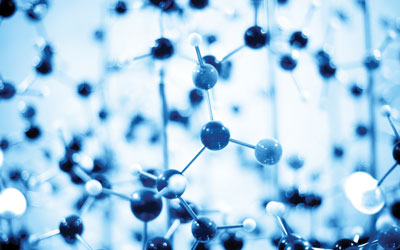
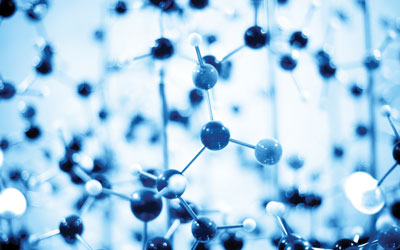
The particles, which may consist of aggregated proteins, and/or components shed from process materials or container closure systems, can directly impact on the efficacy and immunogenicity of a drug product. Also, they often act as nucleation sites for further protein aggregation and/or lead to the development of larger particles by agglomeration. Measuring the size and concentration of subvisible particles within a formulation is an essential precursor to their effective control, and of growing importance as the industry works towards ‘zero defect’ and ‘essentially particle-free’ products. In this article we consider requirements for subvisible particle measurement within the context of current regulatory expectations, and more broadly, review the technology available to meet them.
Analytical instrumentation for the biopharmaceutical industry continues to develop to meet evolving needs, and new tools are being commercialised on a regular basis. Such innovations allow researchers to use orthogonal techniques for subvisible particle characterisation and to gain the insight needed to formulate safe, stable and effective products.
Investigating the impact of subvisible particles
Subvisible particles are usually defined as being in the size range 0.1μm to 100μm, often above the upper size limit for measurement by size-exclusion chromatography but not sufficiently large to be detectable by eye. Such particles may be proteinaceous (aggregates or proteins adsorbed onto other contaminants), silicone oil from the syringes, devices and stoppers used during drug delivery, or other contaminants arising from either the manufacturing or drug delivery process.
Aggregation is a common degradation route for proteins, and is a common source of particles in the subvisible range that must be investigated for all products. The formation of protein particles can have the undesirable effect of reducing product efficacy and also has significant potential to cause adverse side effects. Such effects depend on the drug delivery method and the size and type of aggregates involved, but may include: local reaction, embolism and/or immunogenicity. Immunogenicity is considered a particular problem since it may lead to a fraction of patients becoming ‘secondary non-responders’ due to the formation of neutralising antibodies. This effect can threaten the success of ‘miracle drugs’ and can pose a severe threat to patient treatability.
Current US Pharmacopoeia (USP) specifications include numerical limits for particles >10µm and >25µm in size. These limits are associated with concerns about particles blocking capillaries (average diameter around 7µm) upon injection and causing other health issues1. However, as the technology to characterise and quantify particles in the micron and submicron size ranges has become available, it is clear that large numbers of such particles are routinely delivered to patients.
High subvisible particle counts can result from shedding by process equipment or particles that may be present in components used for drug delivery – IV bags or syringes – or may even be produced by mechanical stress if the product is mishandled during transport. These particles are not routinely filtered out as part of the drug administration process but rather delivered along with the drug. Even with the 0.22 micron in-line filters used for intravenous administration, a large fraction of the submicron particles can readily pass through and be delivered to the patient.
In summary then, developing knowledge of the provenance and impact of subvisible particles is a vital strategy for engineering stability and safety into a product. Indeed, better characterisation and understanding of the role of subvisible particles has the potential to be a cost-effective strategy for developing stable and effective products with the added advantage of offering opportunities for interventions early in the development process.
The regulatory perspective
Evolving understanding of the importance of subvisible particles and their role in triggering immunogenic responses is evident from the requirements of pharmacopoeial chapters USP <788>, Ph. Eur. 2.9.19, and the more recently released USP <787>, which came into effect in 20142,3,4. The latter relates specifically to protein therapeutics and is associated with general information chapter 17875.
The latest guidance reflects the growing concern about particles in the 0.1µm-to-10µm size range and recommends the determination of particle concentrations for the >2µm and >5µm fraction, in addition to the enumeration of particulates in the >10µm and >25µm size ranges. It also calls for assessment of the range and levels of particles in the 2µm-to-10µm range both initially and over the course of a product’s shelf life. Particle concentration limits are included for the larger size ranges, but the new guidance also permits the establishment of product-specific limits. In addition, there is a useful reduction in required testing volumes, with the new chapter allowing for volumes as low as 0.2mL. This not only saves on product costs but also enables the determination of particle count variability from vial to vial or syringe to syringe.
However, beyond these specific requirements it is clear that the regulatory drive is for sufficient subvisible particle characterisation to demonstrate the necessary understanding and control of product quality, within the constraints of current analytical capabilities. For example, the new FDA guidance highlights the need to distinguish silicone oil from proteinaceous, inherent or intrinsic particles, and in addition states that “as more methods become available, sponsors should strive to characterise particles in smaller (0.1-to-2 microns) size ranges.”6. This wider investigative requirement is echoed by the EMA Guidelines7 which state that “[the] formation of aggregates, subvisible and visible particulates in the drug product is important and should be investigated and closely monitored on batch release and during stability studies. In addition to the pharmacopoeial test for particulate matter, other orthogonal analytical methods may be necessary to determine the level and nature of particles.”
So while formulators and quality control groups may understandably look for clarity with regard to regulatory requirements, the reality is that the issue of subvisible particles requires rigorous systematic investigation. Subvisible particle characterisation requirements extend to particle size, number, shape, optical properties and chemical identification, and increasingly includes submicron particles.
Establishing the analytical toolkit
Both USP <787> and <788> recommend the technique of light obscuration (LO) for the sizing and enumeration of subvisible particles. LO instrumentation measures particle size and number on the basis of the blockage of light by individual particles passing through a light-sensing zone.
More widely used for subvisible particle assessment than any other technique, LO nevertheless suffers from certain limitations when set against the evolving list of requirements in this field. The most important of these limitations is a lack of detailed information about the nature of the subvisible particles it detects. Also, LO has a tendency to undercount translucent particles, such as those composed of protein and polysorbate, and has an upper detection limit of around 18,000 to 38,000 particles/mL, depending on the instrumentation employed. The orthogonal techniques that are now beginning to be used widely tend to be those that directly address these limitations and, in addition, extend information-gathering capabilities in the areas of most importance, creating a more complete picture of a sample’s particle profile.
Flow imaging microscopy
Flow imaging microscopy measures the size, shape and transparency of particles using bright-field images captured as an unfiltered solution passes through a flow cell. The resulting data enable the robust classification and differentiation of particles in solution on the basis of a number of different metrics. The ability to measure directly in the solution of interest is important because studies suggest that filtration can significantly impact results of particle characterisation studies8.
A key advantage of flow microscopy is that images of each particle can be obtained, which allows for further particle characterisation. For example, with digital filtering methods, the technique can distinguish certain relatively larger size (e.g., >5 micron) silicone oil droplets from proteinaceous particles. This is due to the spherical nature of silicone oil particles. Other insights into particle characteristics obtained from imaging include their density and whether they are fibrillar or compact. In addition, with samples containing silicone oil one can observe if particles are composed of agglomerates of protein aggregates and silicone oil droplets.
For these reasons flow microscopy has become a popular workhorse widely used alongside LO; however, it too has an important limitation: for particles smaller than 5µm, the method begins to lose resolution and the ability to accurately differentiate types of particles.
Resonant mass measurement
Resonant mass measurement (RMM) sizes and counts particles in the 50nm-to-5µm size range, on the basis of particle buoyant mass, by measuring changes in resonant frequency as individual particles flow through the measurement zone. The data generated include buoyant mass, dry mass and size. This technique is therefore able to differentiate between silicone oil, which is less dense than water, and proteinaceous particles, in the size range where flow microscopy loses resolution; most silicone oil droplets are smaller than 5 micron. Furthermore, RMM has the advantage of being able to directly distinguish silicone oil droplets from protein particles, and does not rely on post-measurement data manipulations. A caveat is that some particles composed of a combination of protein and silicone oil may happen to be neutrally buoyant and therefore not detected.
These unique advantages make RMM a valued addition to the analytical toolkit, especially for samples that include silicone oil, due to the use of prefilled syringes or other primary packaging components that are siliconised or otherwise treated to improve performance. When used in combination, RMM and MFI allow for the robust differentiation and characterisation of both silicone oil and proteinaceous particles across the wide submicron-to-10µm size range.
Raman spectroscopy
For certain particles in the subvisible region, the preceding techniques fail to provide specific chemical identification. For example, excipients such as polysorbate can degrade and form insoluble particles that are similar in shape and transparency to proteinaceous particles. Imaging techniques augmented by Raman spectroscopy offer effective differentiation in such situations by providing the verification of particle chemistry needed for detailed assessment of the provenance and identity of specific populations.
Raman is well known for its high chemical specificity, and, unlike Fourier transform infrared spectroscopy, is insensitive to the presence of water, enabling the characterisation of aqueous solutions. Unknown materials are typically identified through interrogation of libraries of reference spectra, to which product-specific spectra may be added as required. Automated Raman spectroscopy systems provide efficient particle enumeration, characterisation and sample-dependent identification down into the 3µm-to-5µm size range, even for particle species with closely similar morphology. As a result, although it is not as high throughput as flow microscopy or LO, the technique has proven value for its ability to answer the ‘what is it?’ question that arises so frequently in development, manufacturing and stability programs.
Nanoparticle tracking analysis (NTA)
As the sub-micron fraction of the subvisible particle size range comes under greater scrutiny, techniques that enable the enumeration and sizing of nanoparticles are also increasingly vital additions to the characterisation toolkit. Nanoparticle tracking analysis (NTA) is one such technique.
NTA uses a high-resolution digital camera, a laser and specially designed software to track the Brownian motion of individual particles under a microscope. The resulting measurements are used to generate particle size distributions for particles in the size range of about 50nm to 1000nm, in solution. Each particle is sized individually and within a known sample volume; so the concentration of particles, or particle count, can also be determined. These capabilities provide an immediate solution for the growing requirement to understand the contribution of all size fractions of the subvisible particle size range.
The practicalities of measurement
The preceding discussion highlights how the strengths of a range of analytical techniques are being exploited and combined to meet the analytical requirements of the industry. However, it is important to recognise that many of these methods are relatively new and require substantial expert analytical input at every stage: from method development, through sample measurement, and up to and including the process of data interpretation.
The extended characterisation (as opposed to release) methods associated with subvisible particle characterisation are not currently subject to any regulatory requirement for validation. However, analytical data must always be robust to be meaningful and relevant. (Note that even if particle counts are not related to safety and efficacy in patients, the data may still be required for quality assessments and process control). Furthermore, to fully realise their value, techniques must be easy to apply at every stage of the drug development process. For example, if subvisible particles are not being examined in sufficient detail in the early stage of process development, how will later-stage changes that indicate a problem with scale-up be detected?
This practicality and application of methods is an area where progress is urgently required to address issues associated with:
- Data variability – from analyst to analyst and site to site.
- Sample handling – how should the sample be prepared for analysis to produce results that are as relevant as possible to formulation studies, particularly, for example, in the presence of silicone oil?
- The transfer of particle size standards from one protein to another – a standard developed for one protein is not necessarily meaningful for another.
- Sample size – minimising the volume required for analysis is helpful throughout the formulation workflow, but this must be balanced against the requirement to measure representatively.
- Data interpretation – many techniques produce substantial quantities of information but this raises the question of which metrics are critical in terms of defining performance.
- Data archiving – is it necessary to save every digital image obtained for a sample during analysis by flow microscopy?
Addressing these issues is essential for the development of qualified, validated methods that can be used throughout the drug development process and potentially into quality control.
Looking ahead
Developing a rigorous understanding of the provenance of subvisible particles and their role in product quality, as well as their potential role in initiating or causing harm or loss of efficacy is an important and ongoing goal for the biopharmaceutical industry. Being able to robustly characterise such particles is a prerequisite for progress, and there are a number of techniques that have proven value in this field. The need for researchers to delve deeper into the nature and source of subvisible particles and to develop secure control strategies that mitigate risk creates an ongoing push for advancement in analytical technology. By developing robust methods that can be used across the development cycle, analytical scientists and instrument manufactures can help to accelerate drug development as well as increase product safety.
References
- Christie, M, Torres, RM, Kedl, RM, Randolph, TW, Carpenter, JF. ‘Recombinant Murine Growth Hormone Particles are More Immunogenic with Intravenous than Subcutaneous Administration.’ Journal of Pharmaceutical Sciences. 2014; Vol 103, pp128 – 139
- United States Pharmacopeia, <788>: Particulate Matter in Injections
- United States Pharmacopeia, <787>: Subvisible Particulate Matter in Therapeutic Protein Injections
- European Pharmacopeia, 2.9.19 Particulate Contamination: Subvisible particles, Method 1. Light obscuration particle count test
- United States Pharmacopeia general information chapter 1787: Measurement of Subvisible Particulate Matter in Therapeutic Protein Injections
- Food and Drug Administration (US) Guidance for Industry: Immunogenicity Assessment for Therapeutic Protein Products. August 2014
- European Medicine Agency Guideline on Development, Production, Characterisation and Specifications for Monoclonal Antibodies and Related products. Dec 2008
- ‘Characterization of protein aggregates in suspension and on a filter membrane using Morphologically Directed Raman Spectroscopy (MDRS).’ Malvern Instruments application note. Available for download at: http://www.malvern.com/en/support/resource-center/application-notes/AN150722G3-IDProteinSuspensionAnalysis.aspx
Biographies
John F. Carpenter, PhD, is Professor of Pharmaceutical Sciences at the University of Colorado School of Pharmacy, and a Co-Founder and Co-Director of the University of Colorado Center for Pharmaceutical Biotechnology. His research interests include mechanisms for protein degradation and stabilisation in pharmaceutical formulations, during bioprocessing and in delivery systems, and development of advanced analytical methods for therapeutic proteins. He has worked for several years to define rational strategies for stabilising proteins and vaccines during freeze drying and storage in the dried solid. He has published more than 230 peer-reviewed papers and is an inventor on 30 issued patents. He has received several teaching awards and The Ebert Prize. He is a Fellow of the American Association for Advancement of Science and the American Association of Pharmaceutical Scientists (AAPS) and has received the AAPS Research Achievement Award in Biotechnology. He also is the Organiser for the Colorado Protein Stability Conferences.
Amber H. Fradkin, PhD, received her PhD in Chemical Engineering at the University of Colorado at Boulder. Amber currently holds the position of Associate Director at KBI Biopharma where she manages the Particle Characterization Core which specialises in analytical methods for quantifying, characterising and identifying submicron, subvisible and visible particulates.
Christine Vessely has over 17 years’ experience in analytical/formulation development, with products ranging from peptides to monoclonal antibodies, therapeutic proteins to vaccines, novels to biosimilars. Areas of expertise include method development, qualification and validation, the development of reference standards, stability strategy and evaluation, and establishment of comparability and/or similarity.