Understanding the effect of API changes in pharmaceutical processing
Posted: 25 February 2017 | John Gamble (Bristol-Myers Squibb), John Jones (Bristol-Myers Squibb), Mike Tobyn (Bristol-Myers Squibb) | 1 comment
For the pharmaceutical scientist, the journey of an active molecule from the end of its synthetic pathway and crystallisation to its ultimate site of action is a fascinating one, and one that is necessary to understand…
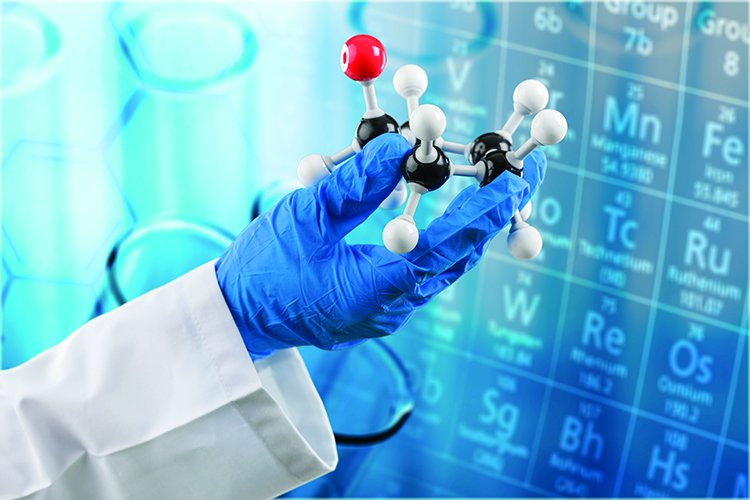
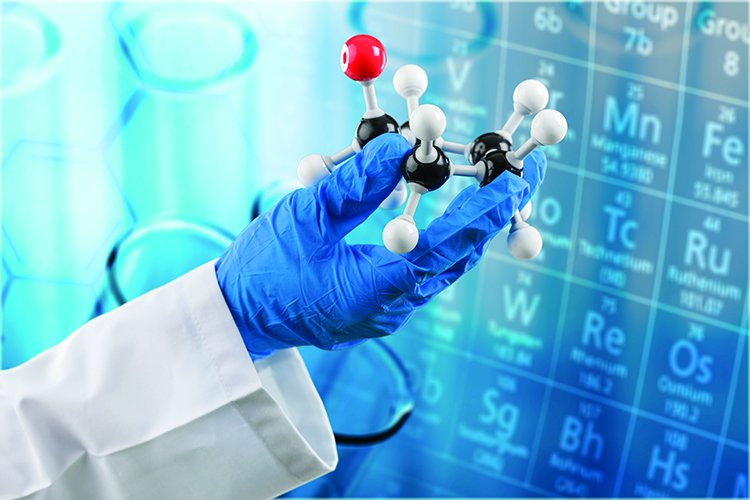
There is, of course, the natural curiosity of the scientist to understand what they are working with, and how the molecule’s journey influences its performance. The regulators who control medicines for the benefit of the patients who take them also require an understanding of what is happening to the drug molecules and particles. These two interests combine in Quality by Design (QbD) initiatives, where scientists and regulators come together to provide a thorough understanding of the manufacturing process of a dosage form, to ensure that it is effective and fit for purpose.
Over the past few years there have been significant scientific advances in understanding how a molecule joins, and is incorporated into, the crystal that is its home until it reaches the gastric intestinal (GI) tract. Despite the fact that the instant of nucleation remains a moment of magic or mystery, the growth of a crystal can be followed, understood and modelled and the process of isolating and drying formed crystals has been closely studied.
The milling process has opened itself to greater understanding in recent years, such that the mechanism and extent of crystal fracture can be followed, and the properties of the resultant particles predicted with greater accuracy. We can characterise the end material, with its single particles, agglomerates or aggregates by size, shape and surface area, and examine them in detail using microscopy techniques. At the end of the particles’ journey to the dosage form we can follow the disintegration of the dosage form and the dissolution of the particles in a range of model media. This data can be combined with other observations to develop models of how drugs will reach the bloodstream and eventually be eliminated.
Many of these processes are amenable to mechanistic modelling, whilst those that are not can be better understood using statistical modelling. Recently, in the United Kingdom, there has been a significant investment in combining all of these models to produce a coherent story from crystallisation to bloodstream. The ADDoPT (Advanced Digital Design of Pharmaceutical Therapeutics, www.addopt.org) initiative brings together large and small pharmaceutical companies, leading academic departments and Government institutions, such as the Hartree Computing Centre, to develop and integrate models to provide an overview of the manufacturing and performance of drug particles. This exciting initiative is already providing valuable insights.
Whether they are required in their billions for successful primary care medicines, or in smaller quantities for more specialty applications, oral solid dosage forms are the preferred method of delivery for the pharmaceutical industry and the patient. They combine scalable manufacture for the supplier with ease of handling for the patient.
There are several routes to a tablet, some requiring fewer processing steps (thus superficially making them more attractive), but it is not clear if ‘equivalent’ processes result in differing extents of ‘mistreatment’ caused to particles during the journey (Figure 1); regimes that are superficially attractive but complex in practice (e.g., direct compression) are limited by such lack of knowledge.
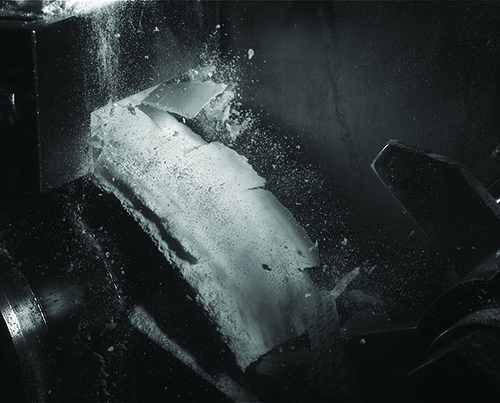
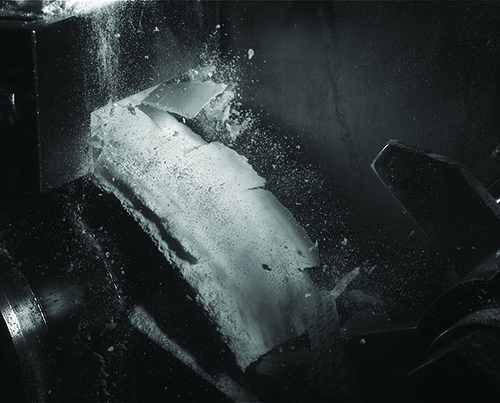
Figure 1: Image of roller compacted ribbon entering milling chamber; a particle’s transition through the manufacturing process stream is seldom a gentle one.
Excipients
One significant gap in the story of a molecule’s journey from solution-to-particle-to-solution arises when the drug particles are mixed with excipients to produce a dosage form suitable for patient administration. Excipients are necessary for the manufacture of solid oral dosage forms. Some excipients form the bulk of the tablet and are there to impart strength and bulk to the dosage form, whilst others help reconstitute the final particles in the GI tract prior to dissolution (disintegrants). Others still are added in small quantities to facilitate particular stages of manufacture (lubricants).
Active pharmaceutical ingredient (API) particles are specific small molecules with very low impurity levels and are often chirally separated with specific spectral features. Excipients are chemically distinct from the API; they are largely organic molecules but can be small molecules, polymers or blends. Excipients are also frequently physically different from an API, being large, relative to many APIs, and microcrystalline or amorphous, compared with the generally crystalline API.
At the end of a particle’s journey it may be possible to discern how the API particles are distributed within a tablet, and whether they are present as agglomerates or not. However, the scale of scrutiny does not allow the size and shape of the API particles to be determined with the detail that could be afforded to unprocessed particles. A recent review covered the potential in understanding the size and shape of unprocessed particles1.
The inability to understand what happens to particles within a blend represents a gap in the understanding of pharmaceutical processes. A particular area of interest in manufacturing technology is the sticking of API to the faces of tablet punches. This process leads to downtime in manufacturing and increasing expense and complexity of tablet manufacture.
Several chemical and physical properties, such as surface area or particle size, can be shown to have some correlation with sticking, whilst methods have been proposed for choosing the contact surface for punch materials. However, the direct correlation between observed particle properties prior to processing and overall sticking potential has not been convincingly made. When making these correlations it is often assumed that the API does not change en route from the initial measurement of its properties to the punch face to which it may attach. However, it is known that ‘fresh’ surfaces are more adhesive, and that increased surface area leads to higher likelihoods of adhesion.
The ability to know whether a particle, or agglomerate, changes during standard pharmaceutical processing could provide new insights into pharmaceutical processing methods and ultimately lead to better end-to-end understanding.
Characterisation challenges
The use of chemical imaging to investigate the distribution of single components with a formulated sample have previously been reported2. However, due to limitations in the optical resolution of such systems, the individual particle sizes cannot be directly measured; pixels often contain more than one of the constituents. Pixels are instead colour coded to indicate the relative concentration of each constituent, thus enabling identification of ‘domains’ (areas of high component concentration). The relationship between domain size and particle size can be affected by multiple factors such as homogeneity, aggregation and morphology.
The challenge of characterising the primary particle characteristics of single components within multi-component systems has recently been addressed through the application of image-based particle characterisation with integrated Raman capability. This approach enables the characterisation of particles in terms of both size and shape. Utilising the Raman probe, the components within a blended sample can be sub-classified in terms of their chemistry, thus enabling the actual particle size distribution of individual components to be determined rather than the domain size.
Examples of this approach have recently been reported; Gamble3 demonstrated the process-induced attrition of a formulated API. It was demonstrated that blending and cone milling process steps had little impact on the primary particle size of the API, whilst a powder feed system, associated to a roller compactor, was observed to significantly reduce particle size. Such attrition could impact the processability of the material, both positively and negatively. This work highlighted that the powder feeding step, a hitherto overlooked sub-process, has a significant impact on the API/blend properties, and therefore requires consideration when selecting sources of process variation.
In addition to size, changes to the particle shape were also investigated. The combination of the two datasets provides insight into the attrition mechanisms within the unit processes. For the milling process, minor shifts in both size and shape could suggest a surface abrasion mechanism where the elongated particles undergo ‘chipping’. For the powder feed system, however, more significant shifts in both size and shape were proposed to suggest a bulk fracture mechanism where the particles undergo more complete fracture.
The work not only demonstrates that the input API size was impacted by the process, but that by characterising the API particle characteristics one could understand the mechanism of the change. This improved understanding of the intermediate API/blend characteristics could be applied to subsequent processing steps, removing the requirement to rely on the input particle characterisation data.
Subsequent work4 utilised the measured API attrition to determine the location of attrition events within the feed system. These efforts applied the understanding of the process/API interaction in order to develop a better elucidation of the unit process and to investigate the impact of varying process conditions on the extent of attrition. The study demonstrated how changes in the feed screw speed could alter the extent of attrition; increased feed rates resulted in increased levels of attrition. This raises an interesting issue; for such unit processes the feed system is often utilised as part of the automated feedback control to maintain the intermediate product characteristics, but if by changing the feed system we alter the blend characteristics, the tool used to control process variation could be a significant source of said variation.
Attrition is by no means the only change that can be induced by such processes. Hoffmann5 combined the above approaches with more traditional chemical imaging in order to demonstrate the dispersion of micronised API, present within a formulation in the form of cohesive aggregates. The use of jet-milling is commonplace for APIs with low solubility. The process results in very fine particles which are commonly cohesive/adhesive in nature and thus can be challenging to disperse uniformly. However, the study observed that the previously investigated powder feed system was able to disperse the particles, leading to a homogeneous blend, whilst not changing the primary particle size of the API.
The aforementioned studies highlight that materials are prone to change during processing, and when this occurs the input particle characterisation is no longer representative of the material in the process, or in the final dosage form. For such materials a key input characteristic may therefore be the propensity for process induced attrition. A number of particle strength measurements are available; however, this is a field that requires further investigation. In the experience of the authors, examples of ‘highly friable’ particles surviving processes intact, where less friable materials do not, have been observed. This would suggest that we need to consider not only the strength of the primary particles, but the nature of those particles within the formulation.
Conclusion
Tools are available that allow unprecedented access to the knowledge of how particles perform during processing – information that was previously unavailable. This allows knowledge, if not understanding, of what happens to particles during manufacture, which may enable a more rational choice of formulation route, and fit with the goals of the Manufacturing Classification System6.
New approaches are providing unprecedented knowledge of the stresses, temperature and other parameters inside the closed box of pharmaceutical processes. By linking this with an outcome (particle attrition) we could combine this knowledge to provide unprecedented access to the inner workings of pharmaceutical systems, with the potential to understand it to a greater degree than ever before.
Biographies
JOHN GAMBLE is a Principal Scientist within the Material Science and Engineering function at Bristol-Myers Squibb. He has been at BMS for 14 years. He is a keen advocate of image-based particle characterisation in the pharmaceutical development environment and has a number of publications in this area.
References
- Gamble JF, Tobyn M, Hamey R. Application of Image-Based Particle Size and Shape Characterization Systems in the Development of Small Molecule Pharmaceuticals. Journal of Pharmaceutical Sciences. 2015;104(5):1563-1574
- Sasic S, Kong A, Kaul G. Determining API domain sizes in pharmaceutical tablets and blends upon varying milling conditions by near-infrared chemical imaging. Analytical Methods. 2013;5(9):2360-2368
- Gamble JF, Hoffmann M, Hughes H, Hutchins P, Tobyn M. Monitoring process induced attrition of drug substance particles within formulated blends. International Journal of Pharmaceutics. 2014;470(1-2):77-87
- Gamble JF, Dennis AB, Hutchins P, Jones JW, Musembi P, Tobyn M. Determination of process variables affecting drug particle attrition within multi-component blends during powder feed transmission. Pharmaceutical Development and Technology. 2016:1-6
- Hoffmann M, Wray PS, Gamble JF, Tobyn M. Investigation into process-induced de-aggregation of cohesive micronised API particles. International Journal of Pharmaceutics. 2015;493(1-2):341-346
- Leane M, Pitt K, Reynolds G, et al. A proposal for a drug product Manufacturing Classification System (MCS) for oral solid dosage forms. Pharmaceutical Development and Technology. 2015;20(1):12-21
Thanks for sharing your thoughts and information on micronization of powder formation.