Life sciences flow metrology challenges
Posted: 14 December 2020 | Dr Tracy Brown (TÜV SÜD National Engineering Laboratory) | No comments yet
To realise quality, yield and cost benefits, there is a clear need to develop expertise and capability in the flow measurement and characterisation of pharmaceutical powder and fluid flows within continuous manufacturing processes. Dr Tracy Brown from the TÜV SÜD National Engineering Laboratory in the UK has investigated potential flow measurement requirements within life sciences. Here, she shares their initial findings.
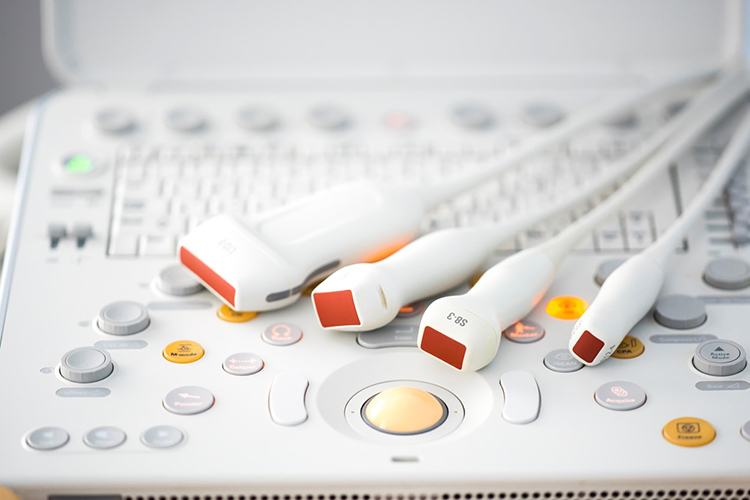
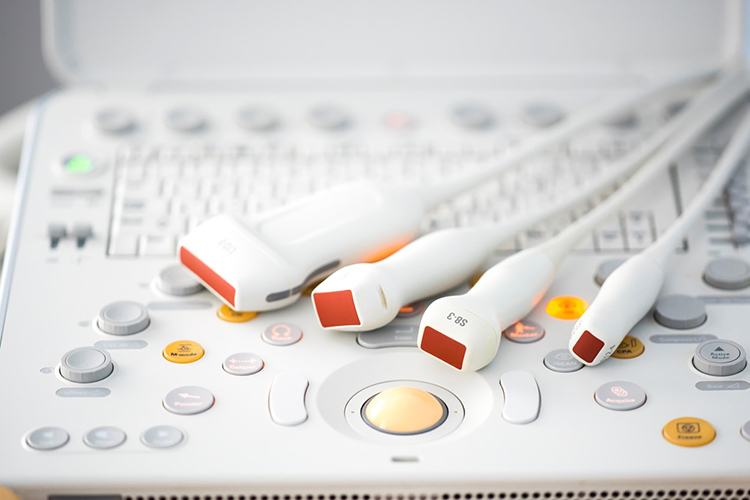
LIFE SCIENCES is evolving rapidly and innovation is forging a path towards personalised medicine and achieving the ambition to live longer, healthier lives. Likewise, disciplines such as biotechnology and medicine have been knocking on the door of metrology for some time to help find solutions for some of the major healthcare challenges we face. ‘Classical’ metrology is continually tasked with extending measurement ranges and reducing measurement uncertainties. National Measurement Institutes (NMIs) establish and maintain measurement standards at the highest level of accuracy, helping to promote fair trade, a safe environment, productivity and products of high quality and reliability. A key role of NMIs is to undertake R&D to develop measurement technology and solutions for the emerging needs of industry.
We have worked in conjunction with the Institute of Measurement and Control to investigate potential flow measurement requirements within life sciences. This was part of the UK National Measurement System’s R&D programme and is also supporting wider academic research. In defining the recommendations made, multiple key stakeholders from academia, industry, healthcare providers, NMIs and life sciences were consulted to provide a wide range of perspectives.
Flow measurement challenges in continuous pharmaceutical manufacturing
Continuous manufacturing (CM) has the potential to transform medicines manufacturing, bringing compelling advantages to patients, industry and regulators through reduced production costs, faster production times and smaller physical and environmental footprints; together with improved quality, scalability and flexibility. The adoption of continuous flow manufacturing within the pharmaceutical industry brings technical flow measurement challenges that manifest from bench/lab, pilot and scale-up, to clinical trial and commercial supply chains. For example, typical issues experienced when dealing with powders include their failure to discharge reliably from hoppers and silos and their unpredictable flow inside feeders, dosing and packing machines. Powder flow behaviour can therefore affect manufacturing efficiency and product quality through interruption to the manufacturing process, while corrected flow restrictions and stoppages may lead to unwanted variations in pack weight and product performance.
Granulation (or agglomeration or pelletisation) is the process of enlarging small particles into coherent and stable masses (granules) in which the original particles are still identifiable. Its purpose is to improve the properties and form of the final product. While both dry and wet granulation methods exist, continuous wet granulation is used more commonly in tablet manufacturing to improve the flow properties of complex powder mixes, to ensure reproducible flow and consistent tablet weight.
Continuous direct compression is another evolving technique used in the preparation of oral solid dosage (OSD) products with potential benefits due to its simplicity. Direct compression is ideal for powders that can be mixed well, with good flowability. It is a popular choice, as it provides the shortest, most effective and least complex way to produce tablets as the active pharmaceutical ingredients (APIs) are blended with the excipient and a lubricant before being compressed.
Investigation of powder flow properties and their relationship to particle morphology (shape and size) will improve our understanding of how particle properties impact bulk powder behaviour under various processing conditions and allow their manipulation to achieve optimum performance in OSD production. Once tablets are compressed, it is then important to ensure that homogenous enteric coating suspensions flow into spray guns in the coating machines or fluid-bed coaters to prevent coating segregation and gun blockage.
Flow metrology is therefore essential throughout all stages of OSD production; but it must be the right metrology, capable of identifying what constitutes good and bad flow. Therefore, there is a need to develop fundamental flow metrology infrastructure and standards to support the adoption of advances in pharmaceutical production. Investment is also required to develop solutions using process analytical technologies for the inline flow measurement of drug powders and fluids, to improve quality and yield in pharmaceutical production.
Non-invasive vascular flow and haemodynamic assessment flow measurement challenges
Cardiovascular disease is the leading cause of mortality globally.1 The measurement of blood flow and tissue perfusion is critical to diagnosis, risk stratification, monitoring and disease treatment. More specifically, there is clinical impetus for identifying reliable, accurate and precise non-/minimally invasive methods for assessing haemodynamics, as well as the systemic blood flow of both large vessels and microvasculature, the latter being important in regulating local tissue perfusion and blood-tissue exchange.
Accurate and time-saving non-invasive haemodynamic monitoring is an aspiration of modern cardiovascular metrology. This pivotal area of clinical research and practice demands innovative technologies to reduce complications, as well as improve morbidity, mortality and the profound socio-economic burden associated with cardiovascular diseases. For example, there is currently a lack of understanding of the pathophysiology of heart failure with preserved ejection fraction (HFPEF) and the precision and accuracy of existing tools for non-invasive measurement of systemic vascular flow and haemodynamics. Given that about half of total heart failure cases are due to HFPEF, a statistic which is rising, better understanding of the pathophysiology of this less-well recognised form of heart failure is vital.
Disciplines such as biotechnology and medicine have been knocking on the door of metrology for some time to help find solutions for some of the major healthcare challenges we face”
Presently, angiography is the gold standard for blood flow quantification. However, it is an invasive procedure involving catheterisation, which poses risks for the patient. Current non-invasive options are limited by their exposure to ionising radiation, temporal and spatial resolution and accuracy, with some methods deviating by up to 20 percent from the results of catheterisation.
According to UK Research and Innovation (UKRI), imaging is now the dominant form of analysis for molecules, cells and tissues across the life sciences.2 Developments in bioimaging tools and techniques may provide insight into the pathophysiology of poorly understood conditions such as HFPEF and lead to better detection, monitoring and therapeutic intervention. Furthermore, improved early detection and treatment of disease through enhanced non-invasive medical imaging methods falls within one of six major societal challenges in the next 10-20 years wherein medical and biological engineers can contribute to solutions, as identified by the American Institute for Medical and Biological Engineering (AIMBE).3
There is an evident role for the inclusion of flow metrology in the R&D of new and evolving diagnostic and therapeutic innovations. This will improve quality, reproducibility and comparability through the provision of reference procedures, materials and data, and deliver traceability to standards. Currently, clinicians rely predominantly on qualitative assessment of imaging scans as the basis for making diagnoses and complex decisions regarding treatment regimens. Only through building capability to make quantitative measurements with non-invasive imaging modalities, reproducible and traceable to relevant new standards, will the necessary assurance be provided to doctors and patients in the accuracy of diagnoses and more personalised therapeutic decisions.
The evaluation and reduction of measurement uncertainty will also be essential to reducing the costs of repeat testing. This also has the potential to enable detection of the signs of disease at an earlier stage in its development. Improving and validating emerging imaging techniques through the routine use of data will therefore enable their translation from a research tool to clinical use.
Presently, the metrological traceability of quantitative diagnostics is underdeveloped, which is limiting the translation of novel technologies to the clinic. Therefore, research focused on developing traceable and reliable procedures for improved imaging resolution, sensitivity, quantification and discriminating power is needed. The increasing complexity, and thus cost, of health metrology demands emphasises the need for a collaborative R&D approach to developing capability involving healthcare providers, NMIs, academia and industry.
Medical imaging
Ultrasound and magnetic resonance imaging (MRI) are non-invasive, non-ionising imaging techniques with potential application in understanding the role of blood flow dynamics in health and disease. These imaging techniques are used widely for quantification of blood velocity and related quantities. However, the clinical exploitation of existing and new haemodynamic measurements using these modalities is dependent upon the development of measurement standards (flow standards) to deliver reproducible imaging results for research, diagnosis and treatment.
The adoption of continuous flow manufacturing within the pharmaceutical industry brings technical flow measurement challenges that manifest from bench/lab, pilot and scale-up, to clinical trial and commercial supply chains”
Diagnosing and treating cardiovascular diseases is increasingly reliant upon quantitative blood velocity measurement to improve objectivity in clinical decisions across imaging centres and geographies. Standardising the use of medical imaging through the development of experimental and synthetic flow phantoms and documentary standards and guidelines will bridge the existing gap by moving towards traceable, accurate and reproducible measurement. In turn, this will bring diagnostic confidence, earlier intervention, personalised therapeutic decisions and enhanced patient outcomes.
There is a need to establish a flow metrology infrastructure to support the optimisation and innovation of ultrasound, MRI and other medical imaging systems. This will result in lower manufacturing costs and improved time to market and, ultimately, lead to better performance and accuracy of imaging systems. Furthermore, standardised flow phantoms should act as enablers of increased uptake of new imaging technologies among clinical and research communities.
Looking forward
There is a clear need to develop expertise and capability in the flow measurement and characterisation of pharmaceutical powder and fluid flows within a continuous manufacturing environment to realise quality, yield and cost benefits. Also, within the clinical setting, non‑invasive cardiovascular flow and haemodynamic assessment is currently underdeveloped. One approach to tackling this is through improvement in the quantification, traceability and reproducibility of medical imaging, vital to accelerating its clinical utility.
Delivering advances in flow measurement capability within these crucial areas will positively impact on the vision of the healthcare and pharmaceutical sectors to transform the prevention, early diagnosis and treatment of chronic diseases and ensure the healthy ageing of individuals.
About the author
Dr Tracy Brown is the Head of Life Sciences and Healthcare at TÜV SÜD National Engineering Laboratory, a provider of technical consultancy, research, development, measurement and testing. Part of the TÜV SÜD Group, the company is also a global centre of excellence for flow measurement and fluid flow systems and is the UK’s Designated Institute for Flow and Density Measurement.
References
- Cardiovascular diseases (CVDs) [Internet]. Who.int. 2020 [cited 1 December 2020]. Available from: https://www.who.int/news-room/fact-sheets/detail/cardiovascular-diseases-(cvds)
- The UK’s research and innovation infrastructure: opportunities to grow our capability [Internet]. Ukri.org. 2019 [cited 1 December 2020]. Available from: https://www.ukri.org/wp-content/uploads/2020/10/UKRI-201020-UKinfrastructure-opportunities-to-grow-our-capacity-FINAL.pdf
- Medical and Biological Engineering in the Next 20 Years: The Promise and the Challenges. IEEE Transactions on Biomedical Engineering. 2013;60(7):1767-1775.
Issue
Related topics
Analytical techniques, Drug Manufacturing, Formulation, Magnetic Resonance Imaging (MRI), Medical Devices, Production, QA/QC, Research & Development (R&D), Technology
Related organisations
American Institute for Medical and Biological Engineering (AIMBE), Institute of Measurement and Control, National Measurement Institutes (NMIs), UK Research and Innovation (UKRI)