Nanoparticles for drug delivery: next-generation biological drugs to treat inflammatory bowel diseases
Posted: 22 April 2021 | Fabrice Navarro (CEA-Leti) | No comments yet
Nanoparticles offer a promising alternative to conventional drug delivery that allow for more precise targeting and controlled release. Here, Dr Fabrice Navarro discusses the benefits of these nanocarriers and their potential as a therapy for inflammatory bowel diseases (IBDs).
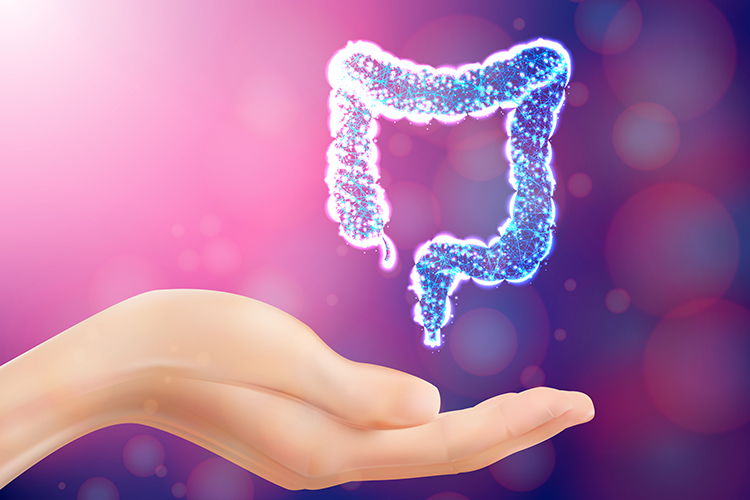
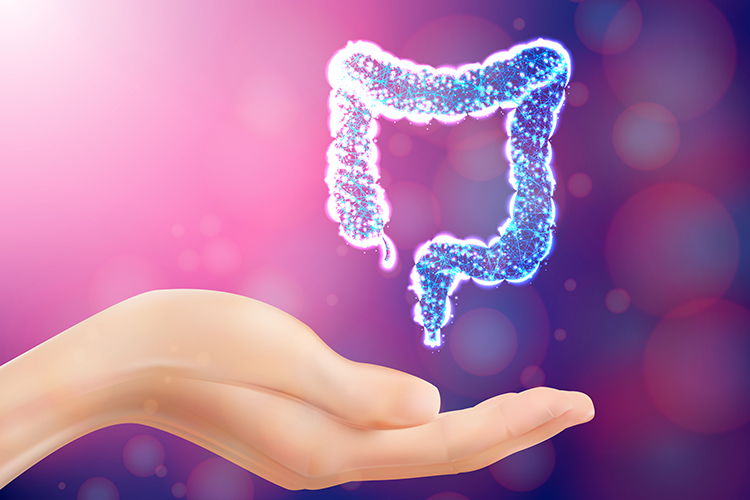
INFLAMMATORY BOWEL DISEASES (IBDs) are chronic, immune disorders of the gastro-intestinal tract with an early onset in life.1,2 Conventional therapeutics like corticosteroids and the first generation of biological drugs, therapeutic antibodies, are effective only for subsets of patients and some patients can lose response over time.3 In light of this, there is an urgent need for more effective therapies.
Recently, chemical inhibitors of Janus Kinases (JAKs) (tofacitinib, filgotinib, upadacitinib), proteins involved in the signalling cascade of numerous cytokines,4,5 have shown promising results in clinical trials,6 but their efficacy has been hampered by their systemic activity and lack of specificity to the cellular and molecular targets.7 JAKs transduce signals from many cytokine receptors of the interleukin and interferon families, as well as from growth hormone and erythropoietin (EPO). This lack of selectivity poses risks and can inadvertently promote serious infections and other side effects.8 For instance, tofacitinib is a potent inhibitor of JAK1 and JAK3 with a moderate potency for JAK2. This chemical inhibitor has demonstrated efficacy as maintenance therapy in a Phase III clinical trial (OCTAVE).9 Moreover, the chemical inhibitors are widely distributed around the body with no selectivity to the inflamed gut. A more specific and selective therapeutic approach with local effects on the inflamed gut is therefore highly anticipated.
Small interfering RNA (siRNA) therapeutics can solve the issue of specificity for the molecular target. This new class of biological drugs has been recently validated in clinic.10,11 Together with the development of RNA vaccines, we can anticipate that RNA therapies as a whole will likely show their potency in the near future for numerous diseases with unmet medical needs. However, these large biomolecules need a delivery method to cross all the biological barriers along their transport toward their cellular targets. Nanocarriers have great potential to solve this delivery hurdle by protecting the siRNA from enzymatic degradation in body fluids, which leads to an extended half‑life, or by promoting diffusion across the plasma membrane.12
Nanoparticles in clinical use
Numerous nanoparticles have been described in the literature for in vivo siRNA delivery purposes, but only very few of them have been translated for clinical use. The most promising carriers for siRNA delivery are those using lipids, which are known to be well tolerated by the organism, whereas polymer cargos present some toxicity and uncontrolled release issues.13
The COVID-19 vaccines race and the resulting innovation based on mRNA delivered by lipid particles will likely unlock the therapeutic use of RNAs for a wide range of biomedical applications in the near future”
In 2021, during the SARS-CoV-2 crisis, lipid nanoparticles for nucleic acids delivery ushered in a new age, benefitting from the pandemic speed to design new mRNA vaccines and promote their use in humans.14 Millions of people received one or two doses of mRNA encapsulated into a derivative of liposomal formulation. These lipid particles present a shell made of a polymer coating and bilayer of phospholipids incorporating specific cationic lipids15 and an aqueous core encapsulating RNA content.
However, these lipid particles, while being relevant for some clinical applications, still present limitations when considering their wide use for tissues and cellular targets other than the liver (Onpattro)16 or activated immune cells at the site of administration (mRNA vaccines).17
In particular, this class of lipid particles has proven inefficient in delivering siRNA across the inflamed gut, likely due to stability issues during their transport through the mucus and/or the intestinal epithelium.18 For this reason, in the European project New Deal (Horizon 2020 grant agreement No 720905) we chose a next generation of lipid nanoparticles with a shell/core structure, where the core is made of a complex mixture of solid and liquid lipids, providing outstanding stability to the particles.19
A lipid-coated drug to treat inflammatory bowel
In the New Deal project, we use lipid nanoparticles with a lipid core as delivery carriers for siRNA therapeutics20 (see Figure 1). We have designed a highly specific siRNA targeting human JAK1, efficient at very low doses with no or minimal off-target effects. We have validated in cells the capability to inhibit the signalling cascade controlled by JAK1 without affecting those controlled by other JAKs (unpublished data, article under preparation). However, selecting the delivery mechanism for in vivo use presented significant challenges given the numerous biological barriers and harsh biological environment in the inflamed gut. For instance, it has already been observed that cationic particles and/or large particles with a diameter above 200nm stick to the mucus and interact with the mucosal content, thus blocking their diffusion.21 Furthermore, particles are exposed to enzymatic degradation (proteases, lipases, etc) into the intestinal lumen and can interact with the microbiota, thus affecting their stability.
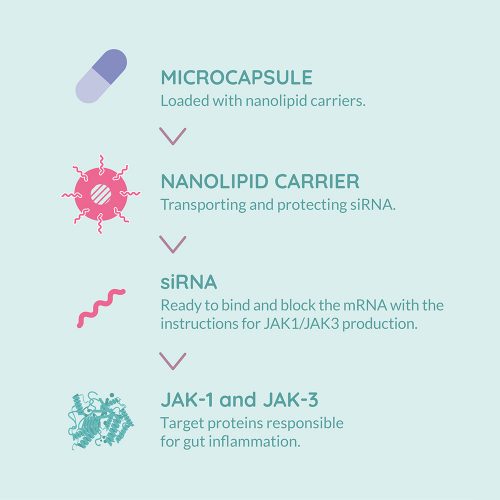
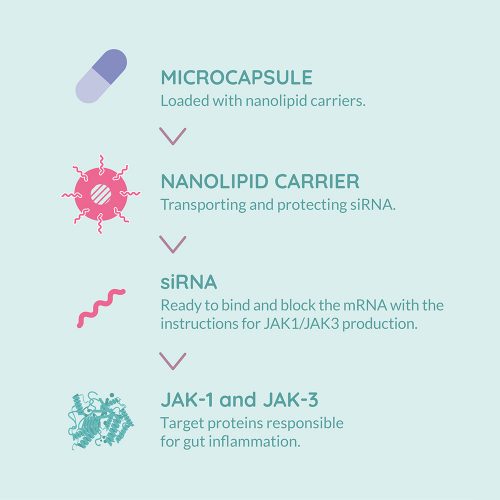
Figure 1: Therapy developed by the New Deal project to treat Inflammatory Bowel Disease (IBD) based on
nanolipid carriers.
In NEW DEAL, we have tested the stability of the nanocomplexes made of siRNA-loaded lipid nanoparticles in several biological media mimicking the fluids encountered along the gastrointestinal transit. We benefitted from the recognised expertise of some partners to test our particle/siRNA complexes in innovative in vitro models at the state-of-the-art level.
First, we tested the diffusion of our particles containing siRNA through several mucus models and properly analysed the results by using fluorescence analytical methods. These models rely on isolated and biosimilar mucus layers with or without mucus-secreting cells. Next, we studied their stability after incubating them in the original SHIME® model (Simulator of Human Intestinal Microbial Ecosystem). The SHIME® is a unique, scientifically validated dynamic model of the complete gastrointestinal tract to study physicochemical, enzymatic and microbial parameters in the gastrointestinal tract in a controlled in vitro setting. The model consists of five reactors which sequentially simulate the stomach (acid conditions and pepsin digestion), small intestine (digestive processes) and the regions of the large intestine.22 Careful control of the environmental parameters in these reactors enables complex and stable microbial communities to be obtained, which are highly similar in both structure and function to the microbial community in the different regions of the human colon.
Finally, we investigated in vitro the transport of particle/siRNA complexes across the intestinal barrier model relying on a co-culture with epithelial and immune cells. After intensive research efforts, we have solved the stability issue and demonstrated that the nanoparticles could diffuse across the mucus and the intestinal cells. More importantly, we have studied the biodistribution of lipid nanoparticles/ siRNA complexes in IBD animal models after their repeated rectal administrations. The dextran sodium sulfate (DSS) model induces an acute self-contained colitis characterised by epithelial cell destruction followed by infiltration of the lamina propria by innate immune cells. We have evidenced in these animal models that the lipid nanoparticles with a lipid core can deliver their siRNA content into a subset of immune cells in the lamina propria (unpublished data, article under preparation). Efficacy after administration of siRNA targeting JAK delivered by lipid nanoparticles is under investigation.
Conclusion
Lipid nanoparticles with a lipid core appear to be a relevant delivery strategy for nucleic acids-based therapeutics since they are composed of biodegradable ingredients with a good control on their safety, presenting high colloidal stability and allowing their long-term storage. Moreover, they can be easily prepared and up scaled by using high shearing processes like high-pressure homogenisation, which are well-qualified processes, thus facilitating their industrial transfer.
The COVID-19 vaccines race and the resulting innovation based on mRNA delivered by lipid particles will likely unlock the therapeutic use of RNAs for a wide range of biomedical applications in the near future. This opens new potential avenues for future regulatory approval and clinical translation of the next generation of lipid nanocarriers, like those we have designed to deliver into the gut siRNA targeting JAK1 in an IBD context.
About the author
Dr Fabrice Navarro obtained his PhD in Molecular and Cell Biology, Physiology and Neurosciences from University Claude Bernard of Lyon in 2007. He joined the CEA Leti, a French research technology organisation, as permanent researcher in 2009 and worked on various projects in the field of nanomedicine. As principal investigator, he was involved in several European initiatives (ERANET Euronanomed projects Target PDT and BIBA, FP7 projects NANOATHERO and NAREB). Since 2016, he is the head of lab of the microfluidic systems and bioengineering laboratory from the health division of CEA Leti, supervising a team of 30 collaborators. He is currently the coordinator of NEW DEAL European project (number 720905), as well as projects dealing with the delivery of proteins or mRNA antigens and organs on a chip. He is co-author of 76 publications and reviewed proceedings as well as co-inventor of 12 patents.
References
- Ananthakrishnan AN. Epidemiology and risk factors for IBD Rev. Gastroenterol. Hepatol., 205-217 (2015)
- Cosnes J, et al. Epidemiology and natural history of inflammatory bowel diseases. Gastroenterology 140, 1785-1794 (2011)
- Gecse KB, et al. Optimizing biological therapy (…), Expert Review of Gastroenterology & Hepatology (2015)
- Zarin AA, et al. Kinase inhibition in autoimmunity and inflammation, Nature Reviews Drug Discovery, Volume 20, Issue 1, January, Pages 39-63 (2021)
- Danese S, et al. JAK inhibition using tofacitinib for inflammatory bowel disease treatment: a hub for multiple inflammatory cytokines. Am J Physiol. Gastrointest. Liver Physiol. 310(3): G155-G162 (2016)
- Sandborn WJ, et al. Tofacitinib as induction and maintenance therapy for ulcerative colitis, NEnglJMed 376(18): 1723-1736 (2017)
- Clark JD, et al. Discovery (…) (JAK) inhibitors for Inflammatory diseases, Med. Chem., 57, 5023-5038 (2014)
- Schwartz DM, et al. JAK inhibition as a therapeutic strategy for immune and inflammatory diseases, Rev. Drug. Discov. 16; 843-862 (2017)
- Lichtenstein GR, et al. Tofacitinib, an oral, small, molecule Janus kinase inhibitor, in the treatment of ulcerative colitis: analysis of an open-label, long term extension study with up to 5.9 years of treatment, Crohns Colitis, 14(Suppl. 1) S100-101, (2020)
- Lam JKW, et al. siRNA versus miRNA as therapeutics for gene silencing, Molecular Therapy – Nucleic Acids, 4 (9), art. no. e252, p. e252 (2015)
- Caillaud M, et al. Small interfering RNA from the lab discovery to patients’ recovery, Journal of Controlled Release, Volume 321, Pages 616-628 (2020)
- Dahlman JE, et al. Nanotechnology for in vivo targeted siRNA delivery. Adv Genet, 88:37-69, (2014)
- Ge X, et al. Rationale and Application of PEGylated Lipid-Based System for Advanced Target Delivery of siRNA, Frontiers in Pharmacology, Vol. 11, 20 (2021)
- Chung JY, et al. COVID-19 vaccines: The status and perspectives in delivery points of view, Adv Drug Deliv Rev,170, 1-25, (2021)
- Wilner SE, Levy M. Synthesis and characterization of aptamer-targeted SNALPs for the delivery of siRNA. Methods Mol. Biol. 1380, 211–224.(2016)
- Buxbaum JN. Oligonucleotide drugs for transthyretin amyloidosis. Engl. J. Med. 379 (1), 82–85 (2018).
- Baden LR, et al. Efficacy and safety of the mRNA-1273 SARS-CoV-2 vaccine, N Engl J Med, 384:403-416, (2021)
- Ball RL, et al. Oral delivery of siRNA lipid nanoparticles: Fate in the GI tract, Scientific Reports 8, (2018)
- Delmas T, et al. Preparation and characterization of highly stable lipid nanoparticles with amorphous core of tuneable viscosity, Journal of Colloid and Interface Science, 360:471-481 (2011)
- https://newdeal-project.eu/
- Maisel K, et al. Effect of surface chemistry on nanoparticle interaction with gastrointestinal mucus and distribution in the gastrointestinal tract following oral and rectal administration in the mouse, J Control. Rel. 197: 48-57, (2015)
- Van de Wiele T, et al The Simulator of the Human Intestinal Microbial Ecosystem (SHIME®). In: Verhoeckx K. et al. (eds) The Impact of Food Bioactives on Health. Springer, Cham. (2015)
Issue
Related topics
Biopharmaceuticals, Drug Delivery Systems, Drug Development, Drug Targets, mRNA, Nanoparticles, Preclinical Research, Research & Development (R&D), RNA, siRNA, siRNA Therapeutics, Therapeutics, Vaccines