Microneedle patch with precise vancomycin drug dose against MRSA skin infections
Posted: 5 January 2022 | Georgios A Sotiriou (Karolinska Institutet), Liv Eidsmo (Karolinska Institutet) | No comments yet
Effective vancomycin concentrations can be delivered locally to methicillin-resistant Staphylococcus aureus (MRSA)-infected sites of the skin with the use of miniaturised needles. This presents opportunities for targeted delivery of medication for focal skin diseases. Here, Georgios A Sotiriou and Liv Eidsmo of the Karolinska Institutet discuss some of them.
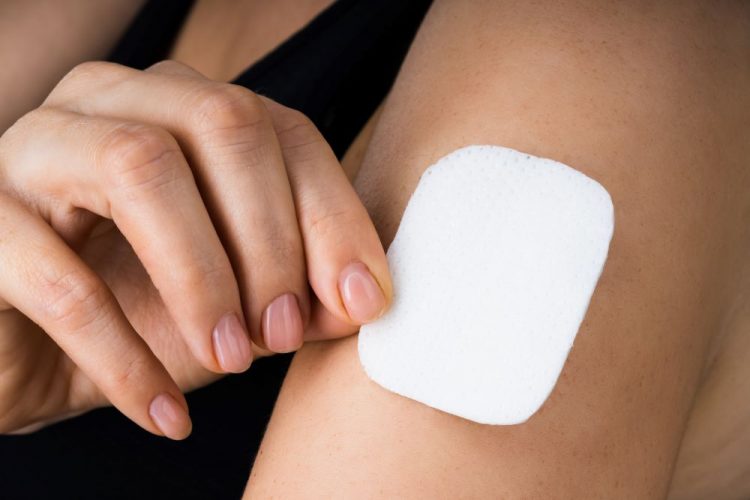
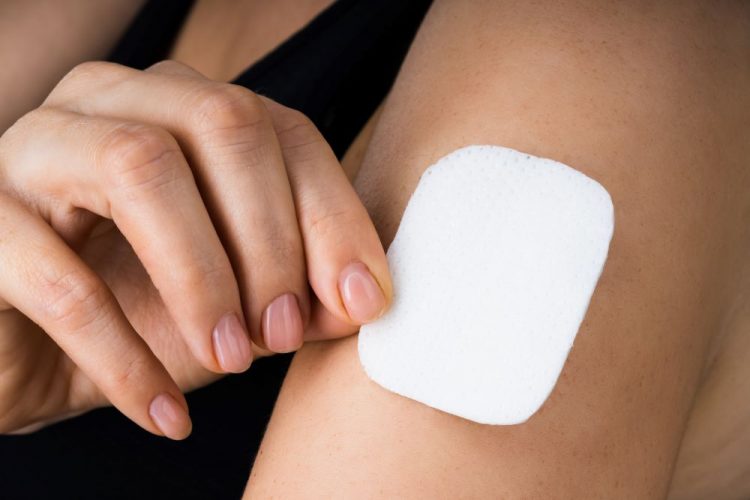
Skin and soft tissue infections (SSTIs) constitute a major healthcare challenge today, particularly when caused by methicillin- or multi drug-resistant Staphylococcus aureus (MRSA), for which conventional antibiotic therapies are ineffective. Such infections may become lethal in immunocompromised patients, such as primary immunodeficiencies or cancer patients receiving chemotherapy. To fight multi drug-resistant infections, systemic treatment with the antibiotic vancomycin is recommended by numerous medical societies globally.1 Vancomycin is a hydrophilic glycopeptide with a molecular weight (MW) of 1.5kDa. The skin barrier stops absorption of molecules with MW >500Da and, due to poor gastrointestinal absorption of vancomycin, intravenous administration is necessary to fight MRSA.2,3 However, intravenous administration of vancomycin requires high doses to achieve effective drug concentrations at the infected skin tissue. These high doses of vancomycin often cause new clinical challenges with side effects that include kidney failure, local pain, thrombophlebitis, and even anaphylaxis or erythema; all the while potentially contributing to resistance development.4 Given the effectiveness of vancomycin against MRSA, topical vancomycin delivery to the skin with precise dosage formulations would broaden the treatment options for localised MRSA infections.
Developing a vancomycin microneedle array
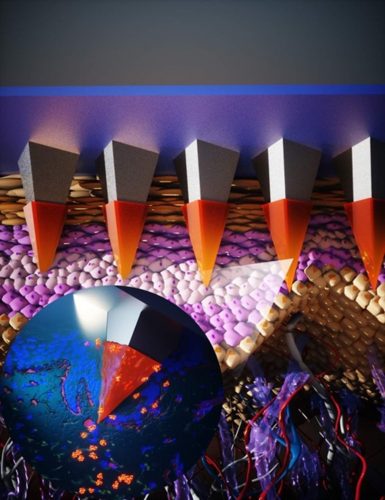
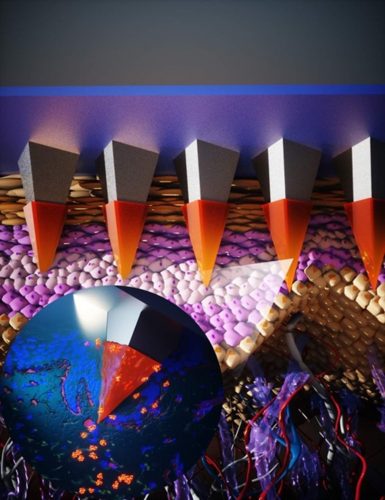
Illustration of a microneedle array with its micron sized needles piercing the stratum corneum (epidermis) to release a drug payload.
To address this important societal challenge, we have recently developed a novel patch consisting of microneedle arrays that can deliver vancomycin locally.5 Microneedle arrays are miniaturised, micron-sized needles that can deliver the drug payload in a highly localised and relatively pain-free manner.6 Despite the existence of several microneedle geometries and architectures, the most common are microneedle arrays composed of a water-soluble polymer incorporating the drug. When the patch makes contact with the skin, the micron sized needles pierce the stratum corneum (epidermis) and the local humid environment inside the skin rapidly dissolves (within a few minutes) the matrix polymer, releasing the drug payload locally. Typically, such microneedle arrays are made by casting the drug-loaded polymer solution on the microneedle molds and subsequently detaching the solid microneedle patch. However, a significant challenge in the successful translation of microneedle technologies to clinics is the poor control over the final drug dosage in the patch. One way to overcome this limitation is to perform the fabrication of the patch in two stages: first the drug-loaded water-soluble polymer is cast to form the needles and then a drug-free polymer is cast as a support material.7 In our work, we advanced this design further by carefully selecting the support polymer material in which vancomycin exhibits poor diffusivity. This design essentially allows for a precise dosage formulation that is only present in the microneedle tips.
To further study the potential of the microneedle patches, we first validated their capacity to pierce the skin. We utilised both thawed porcine skin as well as fresh human skin and validated that in both cases the fabricated microneedle patches can indeed successfully pierce the skin, resulting in the formation of cavities 100-200mm deep, breaching the epidermal-dermal junction. A key benefit is that vancomycin would be primarily deposited and retained locally within the skin, rather than transported through it. This is an essential attribute to obtain the necessary drug concentration to fight local MRSA infections, as the diffusion of the drug through the skin and into the blood stream would lead to sub-optimal drug efficacy and potential development of further drug resistance. This vancomycin retainment in the skin layer may be attributed to the rather large MW of this glycopeptide antibiotic, as it has been observed in literature that smaller drug molecules easily permeate the skin and enter the blood stream.8
The antibacterial efficacy of the developed microneedle patches was studied in vitro with an MRSA growth inhibition model in an agar diffusion assay, validating that the drug is released from the microneedles in its active form and prevented outgrowth of MRSA. Finally, successful vancomycin delivery into the skin was demonstrated with an ex vivo infection model utilising porcine skin, in which the growth of MRSA was inhibited up to three logs, depending on the incubation length and initial amount of MRSA. These results highlight the potential of vancomycin-loaded microneedles in the fight against highly dangerous MRSA skin infections. Our work promotes opportunity for further investigation, not only ex vivo with fresh human skin, but also in vivo with both traditional murine MRSA animal models and humanised murine models with xenografts of fresh human skin.9
What next?
Precise deposition of molecules that do not penetrate the skin barrier opens up several exciting avenues. A natural next step would be to optimise the antimicrobial effect of vancomycin, as our model allows simultaneous deposition of several modalities, or to adapt the drug action to various physiological parameters such as pH or temperature. By co-administering active drugs such as vancomycin together with compounds that provide optimal pH and/or temperature conditions, along with other bactericidal agents, the complete clearance of infection could be investigated. , which could be achieved by loading the drug on nanocarriers.10 A device with multiple antimicrobial mechanisms against MRSA skin infections will not only improve efficacy, but also minimise the emergence of antimicrobial resistance.
precise deposition of drugs in the complex skin microanatomy by microneedles bears great promise to promote tailored treatments for a variety of important clinical challenges”
Given that delivery of a molecule with a high MW such as vancomycin has proven effective against MRSA skin infections in this proof of concept, it seems viable to envision the local delivery of other biological macromolecules such as peptides, proteins and antibodies – the so-called biologics.
One third of the population is affected by skin diseases such as psoriasis, atopic dermatitis, vitiligo, rosacea and acne, presenting a significant burden for individuals and on the health sector. Antibody-based therapies have revolutionised the treatment of severe psoriasis, where the risk of side effects and cost is overcome by the clinical benefit for the patient. Several biologics were recently introduced in the clinic for atopic dermatitis and a promising pipeline of trials for diseases such as vitiligo indicates the number of available drugs will continue to increase. However, the majority of patients present with mild recurrent disease, where the area of involvement does not necessarily justify systemic treatment due to the potential side effects. The relapse in fixed sites can be attributed to long-lived pathogenic immune cells.11,12 In these instances, locally deposited biologics offer great potential to eradicate or modulate the microenvironment to promote deep remission in disease.
Altogether, precise deposition of drugs in the complex skin microanatomy by microneedles bears great promise to promote tailored treatments for a variety of important clinical challenges.
About the authors
Dr Georgios A Sotiriou is tenured faculty and Principal Investigator in the Department of Microbiology, Tumor and Cell Biology (MTC) at Karolinska Institutet (KI) leading the Bionanomaterial Technology Laboratory. The focus of Dr Sotiriou’s research programme at KI is on studying the physicochemical properties of materials made by aerosol processes and then applying this fundamental understanding to tailor sophisticated functional particles and particle-enabled devices for biomedical applications. A specific focus of his lab is against infectious diseases, an under-studied field in nanomedicine.
Dr Sotiriou is contactable at: [email protected]
Liv Eidsmo, MD, PhD is Professor of Translational Skin Immunology and was recruited as Executive Director of the LEO Foundation Skin Immunology Research Center at the University of Copenhagen in 2021. Professor Eidsmo’s research focuses on how T cells residing in the skin drive local recurrence of diseases such as psoriasis and vitiligo.
Professor Eidsmo is contactable at: [email protected]
References
- Liu C, Bayer A, Cosgrove SE, et al. Clinical practice guidelines by the infectious diseases society of america for the treatment of methicillin-resistant Staphylococcus aureus infections in adults and children. Clin Infect Dis. 2011 Feb 1;52(3):e18-55.
- Moellering RC Jr. Pharmacokinetics of vancomycin. J Antimicrob Chemother. 1984 Dec;14 Suppl D:43-52.
- Bos JD, Meinardi MM. The 500 Dalton rule for the skin penetration of chemical compounds and drugs. Exp Dermatol. 2000 Jun;9(3):165-9.
- Shariati A, Dadashi M, Moghadam MT, et al. Global prevalence and distribution of vancomycin resistant, vancomycin intermediate and heterogeneously vancomycin intermediate Staphylococcus aureus clinical isolates: a systematic review and meta-analysis. Sci Rep. 2020 Jul 29;10(1):12689.
- Ziesmer J, Tajpara P, Hempel NJ, et al. Vancomycin-Loaded Microneedle Arrays against Methicillin-Resistant Staphylococcus AureusSkin Infections. Adv Mater Technol. 2021 Jul;6(7):2001307.
- Prausnitz MR. Microneedles for transdermal drug delivery. Adv Drug Deliv Rev. 2004 Mar 27;56(5):581-7.
- Chu LY, Choi SO, Prausnitz MR. Fabrication of dissolving polymer microneedles for controlled drug encapsulation and delivery: Bubble and pedestal microneedle designs. J Pharm Sci. 2010 Oct;99(10):4228-38.
- Singh P, Carrier A, Chen Y, et al. Polymeric microneedles for controlled transdermal drug delivery. J Control Release. 2019 Dec 10;315:97-113.
- Schulz A, Jiang L, de Vor L, et al. Neutrophil Recruitment to Noninvasive MRSA at the Stratum Corneum of Human Skin Mediates Transient Colonization. Cell Rep. 2019 Oct 29;29(5):1074-1081.e5.
- Tsikourkitoudi V, Karlsson J, Merkl P, et al. Flame-Made Calcium Phosphate Nanoparticles with High Drug Loading for Delivery of Biologics. Molecules. 2020 Apr 10;25(7):1747.
- Gallais Sérézal I, Classon C, Cheuk S, et al. Resident T Cells in Resolved Psoriasis Steer Tissue Responses that Stratify Clinical Outcome. J Invest Dermatol. 2018 Aug;138(8):1754-1763.
- Cheuk S, Wikén M, Blomqvist L, et al. Epidermal Th22 and Tc17 cells form a localized disease memory in clinically healed psoriasis. J Immunol. 2014 Apr 1;192(7):3111-20.
Related topics
Antibiotics, Drug Delivery Systems, Formulation, Research & Development (R&D), Therapeutics
Related drugs
Related diseases & conditions
acne, Atopic Dermatitis, MRSA, Psoriasis (PsO), Rosacea, Vitiligo