Shining a light on Raman for microbiological analysis
Posted: 23 February 2023 | Dr Tim Sandle | No comments yet
The detection and identification of bacteria is essential for biopharmaceutical production, the assessment of new antimicrobials and assessing contamination control measures. Developing methods for complex environments is challenging due to interference from the various molecules present. Here, pharmaceutical microbiologist and contamination control expert Dr Tim Sandle explains how Raman spectroscopy enables the assessment of microbial diversity in both solid and aqueous samples.
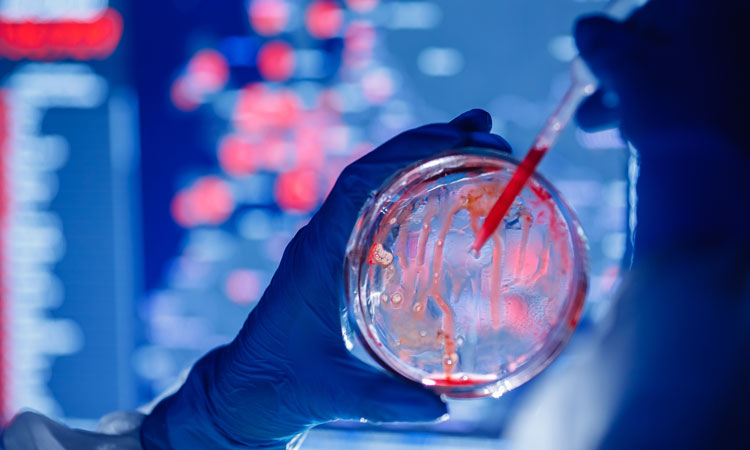
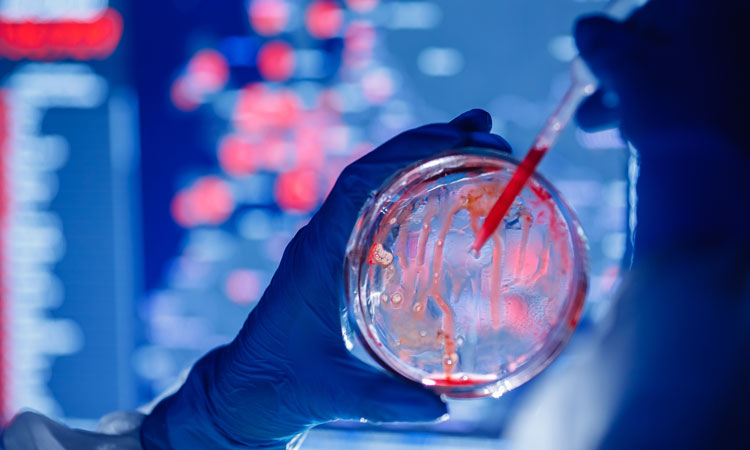
Advances with microbiological methods are providing more accurate and sensitive datasets at a faster rate, relating to bacterial identity, numbers, responses to environments and so on. Recent years have seen progress with applying a long-established spectrophotometric phenomenon – Raman spectra – to advance our understanding of bacterial populations, including differentiating between living and dead cells and interpreting phenotypic variations to different environmental stressors. The first wave of Raman spectroscopic methods for microbiology were developed for clinical areas. The second wave promises useful innovations for pharmaceuticals. This article presents an overview of Raman spectroscopy and details some of the developments of interest to the pharmaceutical sector.
Raman spectroscopy
Raman spectroscopy is a spectroscopic technique based on the vibrational modes of molecules.1 The characteristics of these vibrations provide a ‘molecular fingerprint’ which can be used for identification and counting purposes; in particular, with biomolecules, large sets of information are provided by the vibrational and rotational spectra of different biological molecules.2 When the laser light interacts with molecular vibrations, or other excitations in the molecular system, the energy of the photons shifts either up or down; this is referred to as ‘Raman scattering’. Accurate measurement of these energy shifts enables a detailed analysis of the types of chemical bonds present in the sample. These are characteristics since the wavelength of a photon is changed when it interacts with the sample. The technique is non-destructive and provides information about chemical structure, phase and polymorphy (in essence, different formations), crystallinity and molecular interactions.3 For example, bacteria absorb light with wavelengths of approximately 270nm and certain absorbing biological components will release photons at a higher wavelength (in the form of fluorescence).
Benefits of Raman for microbiology assessment
An advantage of Raman spectroscopy is that, unlike infra-red spectroscopy, it’s not hindered by the presence of water. Other spectroscopic methods either require powders, such as X-ray crystallography, or rigorous sample preparation as with nuclear magnetic resonance. In contrast, Raman spectroscopy offers a more straightforward means to improve microbiological assessments of cell identity and numbers for both dry and aqueous samples.
This is achieved through excitation of the molecules present in a cell and the subsequent capture and record of the resultant inelastic scattering.
The single-cell fingerprint that is produced using Raman contains quantifiable information about its constituent molecules like nucleic acids, proteins, lipids and carbohydrates. Certain spectral regions will be relevant for bacterial fingerprinting.5 For example, a research team discovered that Escherichia coli cells exposed to different environments had distinct Raman fingerprints.6
The Raman approach has also helped microbiologists to better understand the extent of bacteria within a given niche, including those that are unculturable. Raman microspectroscopy, for example, when combined with stable isotope probing, fluorescent in situ hybridisation, and optical tweezers provides a culture-independent approach to study functions of unculturable bacteria within an ecosystem. An example of such an application is for identifying biofilm-forming bacterial strains within a medical setting, which is of relevance for patient infection since many organisms associated with biofilms have strong resistance against antibiotics.7
The application of Raman spectroscopy in the medical field led to its use in pathogen screening; in turn, this has led to a role in drug development, such as time‑lapse experiments to better understand the bacterial growth‑dependent phenomena and metabolic response to novel antimicrobials. While bacteria display cellular diversity, the principle of the Raman spectroscopic approach is that irrespective of the complexity and variety of biomolecules, the physicochemical properties of these biomolecules are interpretable through assessment of their vibrational behaviour. Hence, different bacterial phenotypes can be characterised by unique molecular compositions, which appear as subtle differences in their corresponding Raman spectra enabling interpretation for species identification.
Bacterial identification and enumeration
The primary applications of Raman spectroscopy for bacterial assessment are divided into three areas:
- Identification of different species of bacteria
- Enumeration of bacterial numbers in real time
- Characterisation of the metabolic responses of bacteria to different substrates.
Each of these applications is of current or potential use within the pharmaceutical and life science fields.
Current and potential developments using Raman spectroscopy to interrogate the bacterial diversity of samples from environmental and industrial processes8 are detailed below.
Bacterial identification
Many microbial identification technologies are affected by the bacterial phenotype. Although many phenotype-based systems are reliable and accurate, phenotypic profiles are known to result in uncertain identifications in certain situations, such as when different agars or different environmental conditions are used for incubation. This mimics the phenotypic plasticity that enables organisms to develop different phenotypes in a changing environment and promotes adaptive responses that can lead to organisms taking on different growth characteristics.9 Hence, bacterial cells that live in the same community can exist in different physiological and morphological states according to spatiotemporal variations in environmental conditions. This heterogeneity can sometimes result in inaccurate identifications. A means to overcome this is through characterising the biomolecular profile of cells using Raman spectroscopy coupled with viability staining.
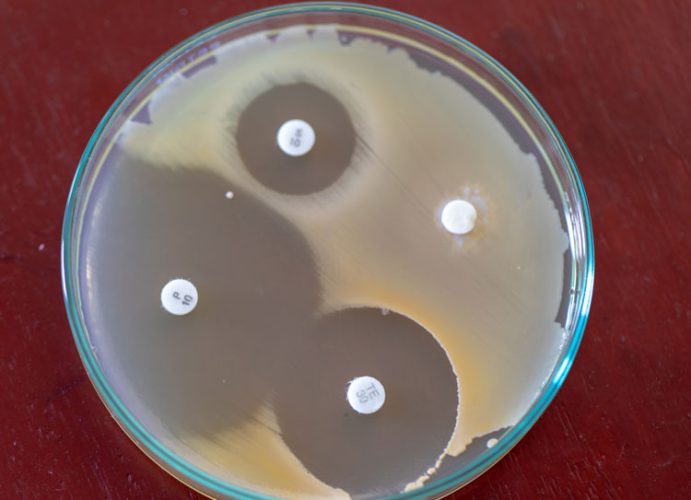
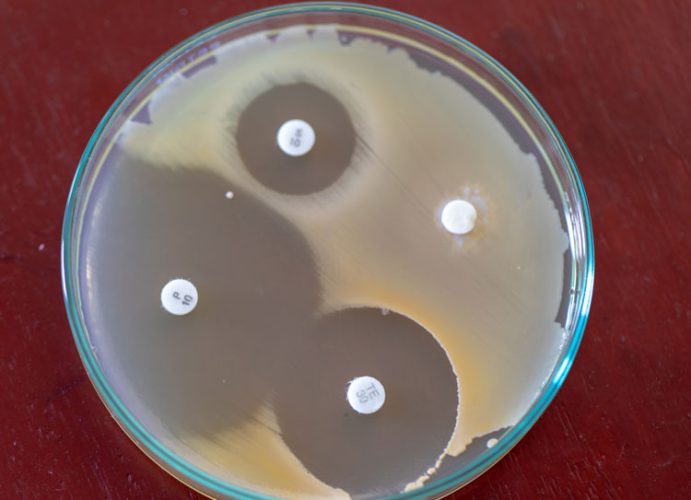
Raman has the potential to accelerate antimicrobial screening for development of new drugs.
Raman has the potential to accelerate antimicrobial screening for development of new drugs.
Here Raman spectra of individual components such as DNA, amino acids and RNA bases, proteins and membrane components, including tryptophan and tyrosine (both of which can produce fluorescence when ultraviolet light is absorbed), can be obtained, as well as individual cells. The extent to which obtaining the spectra of intact, viable cells or dead cells or cellular components is required, depends on the application and whether ‘false positives’ are a concern (such a viable counting). For other types of sample assessment, since tryptophan and tyrosine undergo denaturation when a bacterial population is exposed to a destructive process (such as heat, chemicals or ultraviolet light), a decrease in levels can aid the comparison of live and dead cell populations. This can be useful for the evaluation of selected antimicrobial processes.
Identification is enabled by comparing the obtained Raman spectral signature against a library of known bacteria. Currently, Raman technology is producing isolate-level accuracies exceeding 80 percent, with more accurate profiles obtained from reference cultures. For example, 99 percent accuracy of the signature spectra has been obtained for Staphylococcus aureus, E. coli, Acinetobacter baumannii and Pseudomonas aeruginosa.10
Bioproduction
In relation to identification, understanding phenotypic diversity at the single-cell level provides an important monitoring tool for assessing stress in bioproduction. For industrial processes that require high bioproduction rates, process optimisation calls for stress-tolerant organisms. This is particularly important for commonly used bacteria such as E. coli and Saccharomyces cerevisiae, where alcohol tolerance is an important characteristic.11 Future methods are likely to require samples being collected, labelled, filtered and processed to obtain their spectra. In the longer term, handheld devices could emerge to enable real-time microbial assessments on the production floor.
Antimicrobial screening
Conventional methods to assess antimicrobial sensitivity rely on determining bacterial growth after reaction with a chemical and these require several days for growth, identification and subculture of the organisms in conjunction with an antimicrobial panel at different concentrations. While such assessments are essential in the medical arena (for determining the type of antimicrobial to administer to a patient) and for developing new antimicrobials, the processes are slow. Raman spectroscopy can significantly accelerate the process in both examples.
Understanding sterility
A future-state application of Raman spectroscopy is differentiating between dead and living bacteria following the application of sterilisation processes. This requires discrimination between Raman spectra obtained from organic molecules from bacterial ones within a sample. Several companies are testing the feasibility of the technology for such purposes. At present, optimal bacterial analyses require sample filtering and staining; should Raman spectroscopy be shown to have sufficient detectability (down to one cell) as a non-destructive method then interest will be considerable.
Challenges with Raman spectroscopy
The most obvious challenge with Raman spectroscopy is the need for spectral profiles to obtain an accurate identification. Hence reliability for organism identification relies on the volume of library spectra and how efficiently the system functions for differentiating between species. It will take time to develop libraries that include all of the organisms likely to be encountered within a pharmaceutical setting; however, specific pathogen and objectionable organism screening can provide a more practical application in the short term. The use of Raman spectroscopy as a tool to enumerate bacterial numbers presents a more general laboratory solution.
The first wave of Raman spectroscopic methods for microbiology were developed for clinical areas. The second wave promises useful innovations for pharmaceuticals
Any microbiological method is subject to a degree of variation affecting accuracy, specificity and precision. With Raman spectroscopy, the scattering efficiency is low, which means that the search for, and attempts to interpret, subtle spectral differences can sometimes be problematic, especially where there is background noise caused from other materials (either in the sample or from packaging) and because bacteria produce a relatively weak signal. This can be overcome through instrumentation design via improved and higher signal-to-noise ratios (SNRs). Here, the closer the ratio, the more accurate the identification. As part of method qualification, it will be important to demonstrate that the limit of detection is suitable for the types of analyses required.
How the data is collected and interpreted is also important. Studies have been undertaken using machine learning to improve the interpretative process. For example, a convolutional neural network (CNN) has been developed to classify noisy bacterial spectra by isolate. An alternative means involves surface enhanced Raman spectroscopy, which employs nanoparticles for surface enhancement that increase the Raman Signal.12 A related area concerns how raw Raman spectra data is processed. Such data needs to be subjected to quality control and trimming steps, and the efficiency of these processes affects the final outcome. There are manual and automated approaches to achieve this.
The potential of Raman for microbiology
The current advantages and future potential of Raman spectroscopy as a bacterial identification and enumeration method have been outlined in this article. The strength of Raman spectroscopy relates to the uniqueness of characteristic spectra produced for different organisms and in relation to the technology being rapid, straightforward to use, non-invasive, reproducible, and with the additional advantageous capability to be a real-time monitoring tool.
About the author
Tim Sandle, PhD has over 25 years’ experience of pharmaceutical microbiological research and biopharmaceutical processing. Tim is a member of several editorial boards and has authored 30 books on microbiology, healthcare and pharmaceutical sciences. Tim is Head of Quality Compliance for Bio Products Laboratory Limited (BPL) in the UK and is a visiting tutor at both the University of Manchester and UCL.
References
- Li R, et al. Hand-held synchronous scan spectrometer for in situ and immediate detection of live/dead bacteria ratio, Rev. Sci. Instrum., 2017; 88, Art. No. 114301
- McDermott G, et al. Crystal structure of an integral membrane light harvesting complex from photosynthetic bacteria, Nature, 1995; 374: 517–521
- Wang Y, et al. Multi-point scanning confocal Raman spectroscopy for accurate identification of microorganisms at the single-cell level. Talanta 2023; 254: 124112
- Gerrard DL, Bowley HJ. Instrumentation for Raman Spectroscopy. In: Gardiner, D.J., Graves, P.R. (eds) Practical Raman Spectroscopy. 1989; Springer, Berlin, Heidelberg. https://doi.org/10.1007/978-3-642-74040-4_3
- Huang WE, et al. Shining light on the microbial world: the application of Raman microspectroscopy. Adv Appl Microbiol 2010; 70:153–186
- Teng L, et al. Label-free, rapid and quantitative phenotyping of stress response in E. coli via ramanome. Sci Rep, 2016; 6:34359
- Shakeel M. Surface-enhanced Raman spectroscopy for the characterization of pellets of biofilm forming bacterial strains of Staphylococcus epidermidis, Photodiagnosis and Photodynamic Therapy, 2022; 40: 103145
- Lee KS, et al. Raman microspectroscopy for microbiology. Nat Rev Methods Primers, 2021; 1, 80: https://doi.org/10.1038/s43586-021-00075-6
- Chevin L, Hoffmann A. Evolution of phenotypic plasticity in extreme environments. Philos. T. R. Soc. B, 2017; 372:20160138. doi: 10.1098/rstb.2016.0138
- Ramesh G, et al. Raman spectroscopy as a novel technique for the identification of pathogens in a clinical microbiology laboratory, Spectroscopy Letters,
2022; 55:(8): 546-551 - Jia K, et al. Systematic engineering of microorganisms to improve alcohol tolerance. Eng Life Sci; 2010; 10:422–429
- De Marchi S, et al. Surface-enhanced Raman scattering (SERS) imaging of bioactive metabolites in mixed bacterial populations, Applied Materials Today, 2019; 14: 207-215
Issue
Related topics
Analytical techniques, Drug Manufacturing, Lab Equipment, Microbial Detection, Microbiology, QA/QC, Raman Spectroscopy, Technology