Taking advantage of protein charge properties for challenging separations
Posted: 24 February 2023 | Mareia Frost (Abzena) | No comments yet
In this article, Mareia Frost, Scientific Leader at Abzena, explores how understanding the characteristics of protein‑based drugs, and applying the right techniques, means even the most complex biologics can be effectively separated from potentially-harmful impurities.
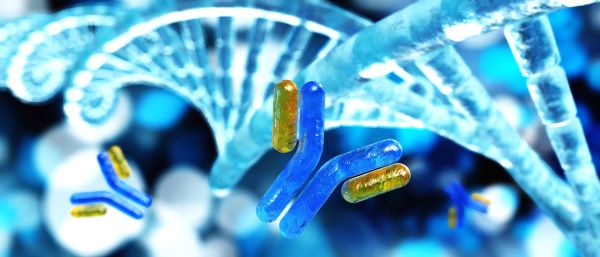
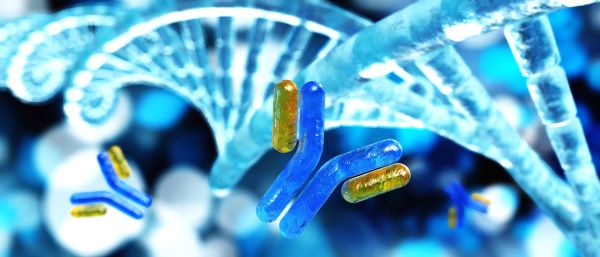
The removal/separation of impurities and ensuring the purity of biologics is essential to the safety and quality of the therapeutic. Not only can impurities lead to adverse effects in patients, but they can also introduce product variability. The risks linked to contamination have led regulatory bodies, including the US Food and Drug Administration (FDA) and the European Medicines Agency (EMA), to implement strict requirements on process characterisation and validation.
To deliver a product of high quality and purity using processes that abide by regulatory guidelines, a robust downstream processing (DSP) strategy is needed.
An ideal downstream process will achieve three aims:
- Process-related impurities (eg, host cell proteins and DNA) and product-related impurities (eg, aggregates and mispaired bispecific antibodies) will be completely removed
- The methods used to achieve purity will enable 100 percent recovery of the target molecule, without impacting activity or stability
- The cost of these processes will be minimal, requiring as few steps as possible.
In actuality, balancing purity, recovery and cost is not a simple task. Choosing methods best able to achieve these goals requires a thorough understanding of the characteristics and properties of the molecule.
Exploiting molecular properties to simplify separation and purification
If the molecule is small, compared with known impurities, filters could be used in order to retain the impurities and allow the smaller target molecule to be collected in the filtrate”
The techniques used to specifically separate and recover the target molecule (drug) from impurities and contaminants in the material resulting from upstream processes depend on the properties of the former. For example, if the molecule is small, compared with known impurities, filters could be used in order to retain the impurities and allow the smaller target molecule to be collected in the filtrate.
Although size-dependent separation methods are commonly used in the DSP of antibody-based therapeutics (eg, centrifugation, size exclusion chromatography, filtration) these will often be used in series with other techniques. Most commonly, these tandem methods will separate the molecule based on its charge.
By understanding the protein charge properties of the molecule, a wide array of tools could be used to separate it from impurities that do not share the same properties.
1. Anion exchange chromatography (AEX)
Molecules with a net negative charge will bind to a chromatography resin (stationary phase) containing positively charged groups (eg, diethyl-aminoethyl groups [DEAE]). Uncharged and positively charged molecules will not bind and will flow through the column. The bound molecules can then be eluted from the chromatography column by changing the buffer (mobile phase) conditions.
2. Cation exchange chromatography (CEX)
Net positively charged molecules will bind to a chromatography resin that contains negatively charged groups (eg, sulphopropyl [SP]). Negatively charged and uncharged molecules will remain unbound when passing the resin and will flow through the column. As with anion exchange, molecules bound to the resin can then be eluted by changing buffer conditions.
3. Hydrophobic interaction chromatography (HIC)
HIC separates molecules based on the hydrophobic differences on the surface of the molecules in the mixture. Molecules with surface hydrophobic pockets (eg, a sequence of non-polar amino acids on the surface) will bind to the hydrophobic ligands of the resin. Hydrophobic interactions are strongly affected by salt conditions due to entropic differences in the ordered structure of water surrounding the molecule, with high salt conditions increasing hydrophobic interactions. By changing buffer conditions to lower salt concentrations, the strength of the interactions with the resin will diminish and the molecules will elute.
As an example, aggregates are generally more hydrophobic than monomers and HIC conditions can be selected to bind the aggregates and allow the monomers to flow through. Although more complicated, similar hydrophobicity differences exist between bispecific antibody (BsAb) homo‑ and heterodimers and can be used to employ HIC purification.
Selecting the right mobile and stationary phase properties
When using these separation techniques, it is important to understand that the charge of a molecule will depend on its isoelectric point (pI) – the pH at which the molecule has a net charge of 0. This means that by changing the pH of the environment (ie, the buffer conditions) the molecules will have a higher or lower net charge that changes independently for each molecule as a function of pH and its constituent amino acids. As a result, the pH of the buffer used in chromatography will impact how strongly the molecules will interact with the resin and how easily they will elute, which can be used to the downstream processors’ advantage.
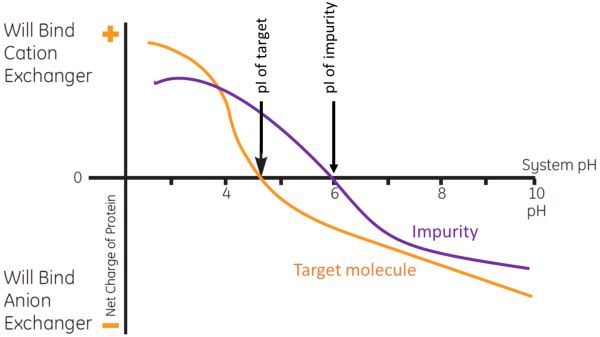
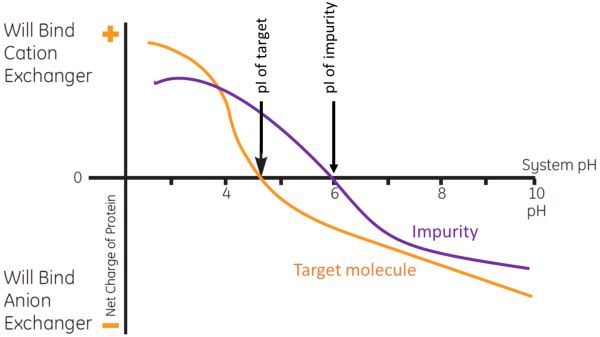
Figure 1: Use of net charge differences between the target molecule and impurity over a range of pH to accomplish separation using AEX.
Figure 1 illustrates the use of net charge differences between the target molecule and impurity over a range of pH conditions to accomplish separation using AEX. At pH 7.0 the target molecule and impurity are both negatively charged; thus, both would bind to an AEX resin. By changing buffer conditions to a lower pH of 5.5, the target molecule will remain negatively charged, but the impurity will now be positively charged. Therefore, an AEX resin at pH 5.5 can be used to bind the target molecule and allow the impurity to flow through. After all the impurities have been washed away, further lowering of the pH below the target molecule’s pI will allow it to be eluted.
The choice of resin will depend on the pI and stability of the molecules to be separated as well as on the localisation of charge throughout the molecules”
Likewise, the ligands of the selected resin can affect the strength of interaction between the proteins and resin. The choice of resin will depend on the pI and stability of the molecules to be separated as well as on the localisation of charge throughout the molecules. Different resins are available, offering the means to benefit from these differences in molecules. These include strong AEX resins (eg, quaternary ammonium), weak AEX resins (eg, DEAE), strong CEX resins (eg, SP), weak CEX resins (eg, carboxymethyl) and hydrophobic resins with ligands of varying carbon chain length (phenyl, octyl, hexyl, butyl, etc).
For downstream processors to purify and recover the target molecule with the fewest necessary steps (reducing cost), a thorough understanding of the target molecule’s properties is needed. This enables optimal buffer conditions and resin to be selected to allow controlled retention and elution of the molecule while thoroughly removing impurities.
Complex molecules bring challenging separations
Advancements in molecular engineering are supporting the production of complex molecules that often offer improved efficacy compared with monoclonal antibody (mAb) therapeutic equivalents. With rising numbers of unique biologics such as BsAbs and fusion proteins entering the development pipeline, downstream processors are facing the challenge of purifying unfamiliar molecules.
Those in DSP can…use their knowledge of the target protein properties and characteristics to purify new, complex molecules”
With expertise and experience, those in DSP can lean on the established principles of separation and use their knowledge of the target protein properties and characteristics to purify these new, complex molecules.
A method that is rising in popularity in BsAb DSP is leveraging mutations that have been made as a tactic to encourage proper heterodimer formation by introducing charged amino acid residues.
The use of these amino acid mutations to promote heterodimer formation often means that the charge properties of the desired heterodimer are different from those of either homodimer.1 This is the case with emicizumab (ART-Ig) and zenocutuzumab (DEKK), for example (see Figure 2).2,3 Consequently, this difference in charge can be utilised during purification steps by favourably using charge-charge interactions in the methods mentioned above.
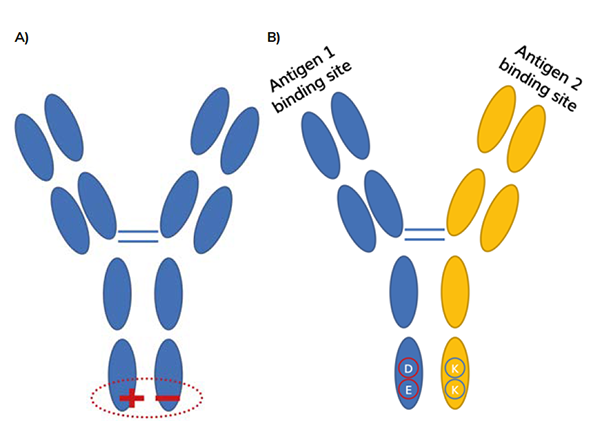
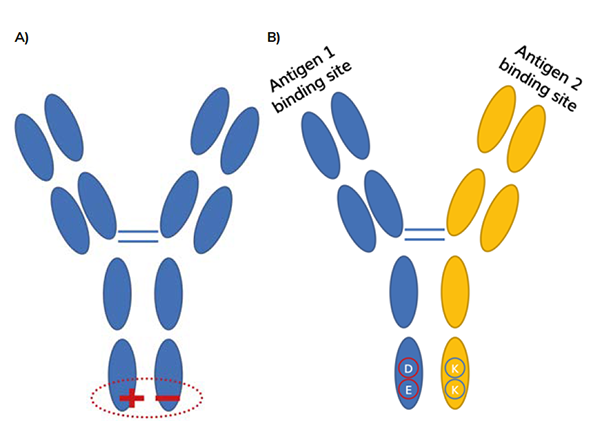
Figure 2: A) Amino acid mutations introduce charge in have chain Fc region to use electrostatic interactions to promote heterodimerisation.1 eg, emicizumab uses this ART-lg platform.2 B) Example: DEKK mutations introduce residues that favour electrostatic interactons between charges.2 D and E amino acids are negative; K amino acids are positive. eg zenocutuzumab (MLCA-128).3
Looking ahead: separation and purification of complex biologics
Years of experience will be needed to know the best approach to take to optimise yield, purity and cost”
As increasingly complex therapeutic biologics enter the development pipeline, reliance on the expertise of downstream developers will rise in tandem. With a myriad of potential techniques that could be applied to purify new protein drugs, years of experience will be needed to know the best approach to take to optimise yield, purity and cost.
However, without extensive characterisation of the molecule in question, taking advantage of the protein’s biochemical properties will not be possible. As a result, it is important to identify support from those that not only offer DSP expertise but transparency between interdepartmental teams to ensure sufficient and open knowledge of the unique attributes of the protein.
About the author
Mareia Frost, PhD is Director and Scientific Leader at Abenza. She has considerable experience with biopharmaceutical operations at various process stages from research and development through commercial in both large and small company settings, having worked at Amgen, Merck, and a start-up. Mareia also has an in-depth knowledge of process theory, having taught at the university level. She has expertise in protein separations with special emphasis on the importance of protein interactions with surrounding surfaces and the role they play on the separation. Mareia holds a PhD in Chemical Engineering from University of Delaware, a MS in Chemical Engineering from University of Washington, and a BSc in Chemical Engineering and Chemistry from University of California at Berkeley.
References
- Kitazawa T, Shima M. Emicizumab, a humanized bispecific antibody to coagulation factors IXa and X with a factor VIIIa-cofactor activity. Int J Hematol. 2020;111(1):20-30.
- Ma J, Mo Y, Tang M, et al. Bispecific Antibodies: From Research to Clinical Application. Front Immunol. 2021;12:626616.
- De Nardis C, Hendriks LJA, Poirier E, et al. A new approach for generating bispecific antibodies based on a common light chain format and the stable architecture of human immunoglobulin G1. J Biol Chem. 2017;292(35):14706-14717.
Issue
Related topics
Biologics, Biopharmaceuticals, Bioprocessing, Chromatography