Raman spectroscopy: an enabling tool for accelerating pharmaceutical discovery to development
Posted: 20 August 2013 | | 5 comments
Raman spectroscopy has emerged as the preeminent analytical tool for a number of applications within drug discovery and development. Advances in the instrumentation, sensor fabrication and data analysis have enabled the wider acceptance of Raman spectroscopy1,2. In discovery, Raman spectroscopy is used to elucidate structural activity relationships3 and to optimise reaction conditions and associated parameters (such as polymorph and formulation screening)4,5 that impact scale-up required for the transfer of drug compounds from discovery to development6,7.
Within pharmaceutical development, Raman spectroscopy has been widely used for non-destructive quantification of actives and excipients8. Moreover, Raman spectroscopy has been proven to be a workhorse as a process analytical technique for process monitoring9,10 (blending, granulation, compression, coating) and understanding of process induced transformation (e.g., effect of excipients, compression force, disintegration of final tablet)11. Raman spectroscopy has also been used to address regulatory concerns over solid state transformations within the final dosage form12. Finally, due to its intrinsic properties such as rapid analysis time, user-friendly interfaces, non-destructive nature, Raman spectroscopy has been used to tackle drug product counterfeit and intellectual property rights issues13.
This review highlights a few representative applications of Raman spectroscopy for in-process characterisation of active pharmaceutical ingredients (APIs) and drug products. Specifically, application of Raman spectroscopy is presented as a tool to monitor the polymorphic state of an API in a slurry, the content uniformity of a blend in a dry mixing process, the end point of analyte dispersion in a suspension and the measurement of a tablet coating process. In each of these cases, Raman spectroscopy has provided in-depth insights into critical quality attributes of the pharmaceutical processes assessed, making the pharmaceutical drug development workflow monitorable in real-time.
API polymorph evaluation
Raman spectroscopy is a powerful technique for characterisation of polymorphic forms of an API. Implementation of in-line Raman probes allows direct real-time monitoring of solid state transformation of an API during the preformulation phase of drug development. As shown in Figure 1, a slurry experiment using 1:1 mixture of Form I and Form II in propanol water mixture at room temperature was performed to determine a stable polymorph of the API. Real-time Raman spectra were collected every two hours for three days (lex = 785 nm, Power = 400 mW, Tcollection = 120 seconds). In order to normalise the spectra and monitor changes over time, first derivative followed by standard normal variate (SNV) was performed on the Raman spectra. Principal component analysis (PCA) of the Raman spectra indicates that Form I (1662 cm-1) converts entirely to Form II (1657 cm-1) within around 20 hours (data not shown) at room temperature, therefore demonstrating that Form II is the more stable form of the API at room temperature.
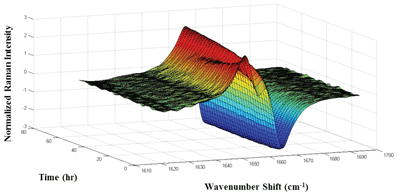
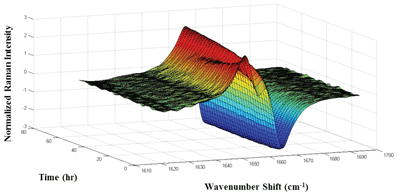
Figure 1
Quantitative determination of components in a dry powder inhaler drug product
The traditional method for determining blend content uniformity of a mixture is to sample the powder followed by off-line measurements. A sample thief is used to sample small volumes of powder from the blender, and they are subsequently assayed using liquid chromatography to analyse the drug product14.This technique is not suitable for rapidly testing hundreds of samples that are manufactured during formulation screening and development, due to time-consuming sample preparation and lengthy analysis time. In addition, it is technically challenging to sample only a few milligrams of powder using a sample thief, a unit dosage for a dry powder inhaler (DPI), without disturbing the blend.
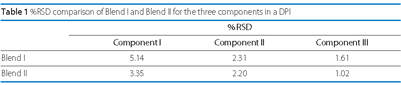
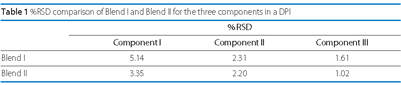
Table 1
In this study, a DPI blending process was used to demonstrate the use of Raman spectroscopy as a robust and rapid screening method to measure content uniformity of the powder blends8. The DPI blend of interest contained three components that have distinct Raman spectral fingerprints, making the system an excellent candidate for quantitative analysis of all the three components. Partial least squares (PLS) calibration models were constructed to quantitate all three components. DPI blends from two intermediate blending processes (blend I and II) were evaluated. Raman spectra were collected from blend I (n = 35) and blend II (n = 80). Figure 2 shows the content uniformity of the batch normalised to 100 per cent for each component. The relative standard deviation (RSD) measured for all three components are five per cent or less for both blend I and II (Table 1). As the blending process continues, the blending %RSD improves for blend I versus blend II, therefore demonstrating that Raman can be a useful technique in determining an end point for blending. In Figure 1, the dotted lines represent the dose content uniformity (DCU) US specification (80-120 per cent)15 of a DPI. The data show that the content uniformity of both blends is within the suggested DCU specification, indicating a uniform batch. If a blend would fail to meet the DCU specification, this method allows one to verify expediently whether it is the blending process that is at issue and correct it prior to making the drug product.
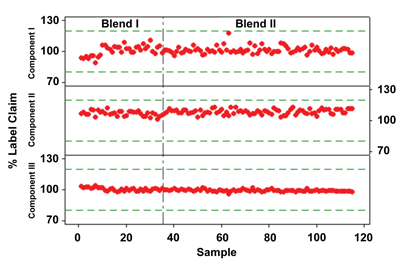
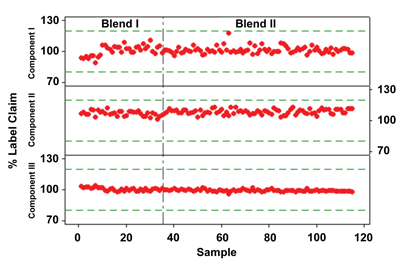
Figure 2
Process monitoring of a nasal spray drug product
Raman spectroscopy inherently has a minimal interference due to water, making the method an excellent candidate to monitor processes in an aqueous formulation such as an aqueous nasal spray. In this study, Raman spectroscopy was implemented to determine the homogeneity of an analyte for a nasal spray product during batch manufacturing16.
The analyte used in this experiment has a distinct Raman spectral region of interest, which does not interfere with other ingredients in the formulation. The PhAT probe was used to collect the Raman spectra from the top of the vessel so that at t = 0 minutes, the Raman spectrum of the drug substance in the mixture was the most intense. Raman spectra were collected every 15 seconds with five second accumulation times. As the analyte is charged into the vessel, the signal is the highest and as the analyte is dispersed, the signal decreases to reach a steady state, implying that the API in aqueous medium is uniformly dispersed. Figure 3A is the 3D plot of the real-time Raman spectra of the analyte signal, which decreases over time. PCA was performed on the Raman spectra after fitting them to second order polynomial baseline correction in order to remove the varying background (Figure 3B).
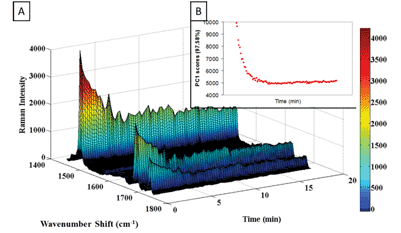
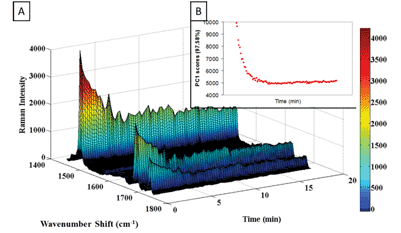
Figure 3
To determine the optimal processing time for analyte dispersion, a running RSD was calculated on every two consecutive principal component 1 (PC1) data points. The steady state of the dispersion process was considered to be achieved when the RSD remained below three per cent for fixed number of minutes.
Tablet coat thickness measurement
Coating processes are important unit operations associated with the manufacture of many solid dosage forms. It serves to impart numerous advantages to a dosage form such as odour / taste masking, physical / chemical stability and modified / sustained release. The thickness and uniformity of coatings provide information on the quality of the coated tablets. Direct measurement of coat thickness is seldom carried out; instead, indirect measurement, such as estimation of coating thickness by weight change, is practiced.
In this study, Raman spectroscopy was used to quantify the tablet coating thickness as a direct measure17. Placebo tablets (normal, circular, biconvex, six millimetres in diameter) made by direct compression were used for coating. Hypromellose contained in the coating formula has a prominent Raman peaks at approximately 1480 cm−1 where aliphatic ether deformation of the polymer occurs18. Acquisition of Raman spectra was carried out at an excitation wavelength of 785 nanometres. Each tablet was placed in a sample holder on a mounting stage at the focal distance of the probe and scanned on both surfaces using a beam size of 300 µm. Acquired spectra were subjected to spectral pre-processing such as Savitzky–Golay smoothing and SNV algorithm in order to remove sampling variations. Raman spectra were correlated against changes in coating thickness as coating progresses. As the coating level increased, the intensity due to the tablet core decreases and the intensity due to the coating increases (Figure 4A). Reference thickness measurements of these tablets were obtained using optical microscopy. Raman spectra were collected from 25 tablets to generate PLS calibration model and independent Raman spectra were used for prediction (Figure 4B). The root-mean-square error of calibration (RMSEC) and root-mean-square error of prediction (RMSEP) are 2.16 and 2.73 µm respectively, indicating the sensitivity and accuracy of Raman technique for the coating thickness prediction. Measured (reference values using optical microscopy) and Raman-predicted coating thickness values indicate no statistical significant difference. Overall, this work demonstrates that Raman spectroscopy is a viable analytical technique to measure coating thickness with similar accuracy compared to that of optical microscopy.
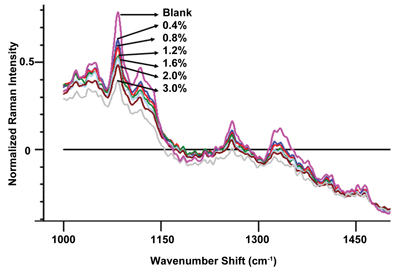
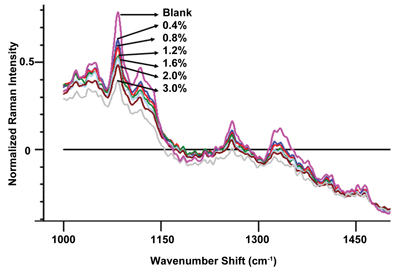
Figure 4A
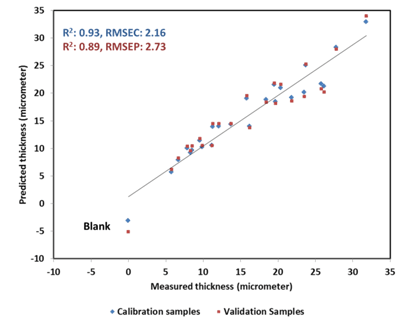
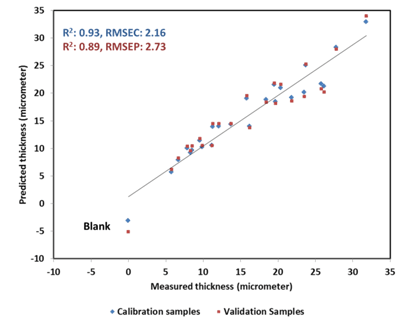
Figure 4B
Conclusion
Raman spectroscopy is a powerful technique that is widely used within drug discovery and pharmaceutical development. Raman spectroscopic methods in conjunction with the application of in-line probes and/or usage of chemometric methods are widely used in a plethora of pharmaceutical applications. In this review, a few examples of in-process monitoring, which resulted in better understanding of the process and product critical quality attributes, were discussed. These examples further demonstrate that applications of Raman spectroscopy in the pharmaceutical industry can lead to significant optimisation of workflows and understanding of in-process manufacturing steps.
References
- Corredor, C.C.; Jayawickrama, D.; McGeorge, G.; Both, D. “Monitoring of blending uniformity: form conversion and fluid bed drying by near infrared and Raman spectroscopy” American Pharmaceutical Review, 2010, 13(1), pp 66-72
- Widjaja, E.; Kanaujia, P.; Lau, G.; Ng, W. K.; Garland, M.; Saal, C.; Hanefeld, A.; Fischbach, M.; Maio, M.; Tan, R. B. H. “Detection of trace crystallinity in an amorphous system using Raman microscopy and chemometric analysis” European Journal of Pharmaceutical Sciences, 2011, 42(1-2), pp 45-54
- Pivonka, D. “Vibrational analysis of structure activity relationships (SAR) in molecular binding Applied spectroscopy” Applied Spectroscopy, 2004, 58(3), pp 323-331
- Heinz, A., Strachan, C.J., Gordon, K.C., Rades, T., “Analysis of solid-state transformations of pharmaceutical compounds using vibrational spectroscopy,” J. Pharm. Pharmaco., 2009, 61(8), pp 971-988
- Xie, Y., Cao, W., Krishnan, S., Lin, H., Cauchon, N.,“Characterization of mannitol polymorphic forms in lyophilized protein formulations using a multivariate curve resolution (MCR)-based Raman spectroscopic method,” Pharm. Res., 2008, 25(10), pp 2292-2301
- Zhou, G.; Guenard, R.; Ge, Z., “Infrared and Raman spectroscopy for process development” From Applications of Vibrational Spectroscopy in Pharmaceutical Research and Development, 2007, pp 185-211
- Yong Z.; “Model for Raman spectroscopic monitoring of process-induced pseudomorph interconversion” American pharmaceutical review, 2009, 12(4), pp 56- 62
- Yonzon, C.R.; Donovan, B.A., “Raman spectroscopic method for content uniformity of a dry powder inhaler,” Amer. Pharm. Rev. 2008, 11(7), pp 70-76.
- El Hagrasy, A.; Chang, S.; Desai, D.; Kiang, S. “Application of raman spectroscopy for quantitative in-line monitoring of tablet coating”, American Pharmaceutical Review, 2006, 9(1), pp 40-45
- Wikstroem, H.; Lewis, I.R.; Taylor, L.S. “Comparison of sampling techniques for in-line monitoring using Raman spectroscopy”, Applied Spectroscopy 2005, 59(7), pp 934-941
- Xie, Y.; Cauchon, N. “Raman scattering as a probe for properties of active pharmaceutical ingredients in tablet formulations” American Pharmaceutical Review, 2012, 15(2), pp 26, 28-31
- Gao, Q.; Lew, A.; Takahashi, L.H.; Cassella, J.V. “An investigation into the morphology of loxapine in a thermal aerosolization process from crystalline to amorphous”Journal of Pharmaceutical Sciences, 2011, 100(4), pp 1407-1415
- Kwok, K.; Taylor, L.S. “Raman spectroscopy for the analysis of counterfeit tablets”, From Infrared and Raman Spectroscopy in Forensic Science, 2012, pp 561-572
- Muzzio, F.J.; Robinson, P.; Wightman, C.; Brone, D. “Sampling practices in powder blending. International Journal of Pharmaceutics”, 1997, 155, 153-178
- U.S. Department of Health and Human Services, Food, and Drug Administration, Center for Drug Evaluation, Research, “Guidance for Industry: Metered Dose Inhaler (MDI) and Dry Powder Inhaler (DPI) Drug Products” 1998
- Pu, Y.; Medendorp, J. P.; Yonzon, C.R. “Real-time monitoring of active ingredient dispersion in a pharmaceutical aqueous suspension using Raman spectroscopy” Journal of Raman Spectroscopy, 2011, 42(11), pp 1994-1999
- Cahyadi, C.; Karande, A.D.; Chan, L.W.; Heng, P.W.S. “Comparative study of non-destructive methods to quantify thickness of tablet coatings” International Journal of Pharmaceutics, 2010, 398(1-2), pp 39-49
- Romero-Torres, S.; Perez-Ramos, J.D.; Morris, K.R.; Grant, E.R. “Raman spectroscopic measurement of tablet-to-tablet coating variability” Journal of Pharmaceutical and Biomedical Analysis, 2005, 38(2), pp 270-274
Biographies
Dr. Chanda Yonzon ([email protected]) is an Associate Principal Scientist at Merck Manufacturing Division. She received her PhD in Chemistry from Northwestern University, Evanston, IL under the supervision of Richard P. Van Duyne. She has extensive experience in vibrational spectroscopic techniques and has applied these techniques to understand several pharmaceutical drug product dosage forms and their processes. She has supported numerous projects in preclinical to commercial and supply stage. She has authored over 25 publications and presented her research at several conferences.
Dr. Atul Karande is currently a Senior Specialist engineer at Merck’s Respiratory product development group, where he is part of the drug product development team. He received his PhD in Pharmaceutics from National University of Singapore. He has thorough understanding of quality by design concept in manufacturing of different pharmaceutical dosage forms. He has supported inhalation and intranasal projects in preclinical to commercial stage at Merck Research Laboratory and Merck Manufacturing Division. Dr. Karande has several original research articles and conference presentations within pharmaceutical sciences. Before joining Merck, he worked at Meggle GmbH as a Technical Application Manager focusing on optimisation of lactose characteristics for improvement of dosage form performance.
Dr. Sai Chamarthy received an MS degree in Pharmaceutics from Duquesne University and a PhD in Industrial and Physical Pharmacy from Purdue University. Dr. Chamarthy is currently an Associate Director at Merck’s Respiratory product development group, where he is part of the drug product development team. Dr. Chamarthy is the current Chair for the Inhalation and Nasal Technology AAPS Focus Group (INTFG) and an executive committee member for the Process Modelling and Simulation Focus Group (PMSFG). Dr. Chamarthy has published over 20 papers in peer-reviewed journals. His research has also led to over 15 podium and 30 poster presentations at various national and international conferences.
Dr. Brent Donovan is an Executive Director leading the respiratory product development department within Merck Research Laboratories in Summit, NJ. He leads a team of scientists that are engaged in analytical and formulation development of dry powder inhalers, metered dose inhalers and nasal spray products. His group has been working on developing novel methodologies for the characterisation of inhalation products as well as supporting numerous projects in all phases of CMC development. He received his PhD in Physical Chemistry from the University of Michigan. Dr. Donovan has several published original research articles within chemistry and pharmaceutical sciences. He previously worked at Schering-Plough for nine years prior to the merger with Merck in 2009.
Please send me a copy of this paper. Thank you
Hi Brian, you need to register here in order to read the full paper. Regards – Nick
please send me this paper
Hi Aydin, you need to register here in order to read the full paper. Regards – Nick
Glad to hear the another used of Raman spectroscopy. This is really a good tool that has been popular in the science industry and now I am happy to know that it is also used to counterfeit drug product. I hope this was gonna be a big help in pharmaceutical industry. Thanks! EnwaveOpt.com