Biomarkers for cancer treatment
Posted: 22 October 2013 | | No comments yet
There is an urgent need to predict which treatment will report the most benefit to a patient with cancer. To that end, scientists are exploring any possible biomolecule in the organism that can mark each individual for its adequate treatment. If achieved, it will open a personalised medicine era.
Introduction
Initial cancer treatments were based on medical experience. Empirically tested drugs were applied to tumours grouped by location, clinical features or size. As the understanding of the disease increased, anatomopathological analysis and clinical phenotype were included as the initial markers for tumour treatment selection. Different treatments were given to different tumour types and both were evolving together along with the discovery of new drugs. Nowadays, more than 200 types of tumours with many more subtypes can be identified, many more drugs, either cytotoxic or targeted, have been developed and a specific treatment is given to a patient according to a combination of clinical features, pathological analysis and molecular markers. However, this assignment is far from clear. For some time, the complexity of assigning an adequate treatment or predicting the individual response to a specific one, even if it is indicated to that type of tumour, has been an issue. Very few tumours are really cured by chemotherapy (for example, most testicular cancers with cisplatin), and in others, the same compound does not work. Why? Even in testicular cancers, around five per cent of tumours are not cured and progress; can we predict which ones? For most tumours and drugs, the whole population shows a relative improvement with some patients showing benefits while others do not. Can we identify these groups? The identification of markers of biological origin, or biomarkers, which enable predicting the response to cancer treatment, has become a research field in itself. A quick look on PubMed shows more than 200,000 entries for cancer biomarkers and more than 30,000 reviews on the topic. There are thousands of publications on every type of biomarker.
There is a clear need to predict the response of an individual tumour to any given drug in order to select the most adequate therapy among the possibilities. There is also a need to identify the degree of toxicity associated to the treatment proposed in order to adapt the dosage to obtain the best benefit / quality of life ratio for the patient. Also, the reduction of treatment costs is an important asset to the biomarker field, i.e. not treating patients with an expensive drug if they will not benefit from it. Given the molecular variability among the tumours of the different individuals discovered by recent whole genome sequestration projects, it seems that every tumour has become a unique entity with unique treatment challenges. This is personalised medicine.
Beyond anatomopathological analysis, which still provides important information about diagnosis, every aspect of biology has been used to provide putative biomarkers, also generating large subfields, high specialisations and new words for the dictionary. Biomarkers have been searched in the genome (genomic, or its variations with non-structural modifications, epigenomic), proteins (proteomic), mRNA (transcriptomic) and metabolic products (metabolomic).
Genomic markers
Cancers harbour mutations, either large chromosomal aberrations or single nucleotide changes. Large alterations can be detected as chromosomes change, gain, lose or translocate. Many sarcomas and leukaemia and lymphomas types are defined by the specific translocation which seems to initiate the tumour. According to this, each translocation also defines the treatment, becoming not only diagnostic but also the marker for treatment. However, in many cases, more advanced biomarkers are needed to identify responders from non-responders. Single gene mutations (either one or several nucleotide changes) are more difficult to identify and require sequencing of the gene. New techniques are also being set up to detect individual nucleotide changes based on variations of differential PCR or real-time PCR and some of the more studied mutations with clinical relevance are established to be performed in an extremely easy and reliable way. For example, in lung cancer, the current first line treatment is determined at the molecular level by the presence of EGFR mutations, K-ras mutations or ALK translocations, all of them causal or driver mutations whose apparition is directly linked to the tumoral phenotype. Other mutations such as MET or ERB2 amplifications or B-ras or PI3K mutations are also being explored. But single mutations can also occur outside of the coding sequences, resulting in differential regulation of the gene. The same can happen with some SNPs providing variations in the nucleotide that are not translated to amino acid change1.
Clinical application of a given biomarker for treatment is also dependent on the treatment alternatives. There is little benefit in applying an exclusion criterion if the patient does not have an alternative treatment. In this regard, treatments and biomarkers seem to evolve together, especially in targeted therapies. Cytotoxic treatments, whose applications are based on experience rather than in molecular mechanisms, are more difficult to predict. These drugs have a mechanism of action that affect the basic cellular machinery; DNA replication, topoisomerases, nucleotide metabolism, DNA-repair, oxidation, etc. Therefore, it is difficult to associate one single mutation / target to resistance or sensitivity to the treatment2-4. In fact, most cytotoxic drugs have many mechanisms responsible for efficacy, from proteins involved in response (i.e.; ATM, ATX, Chk, BRCA1, ERCC1, p53, etc.), to linear pathways altering apoptotic response (i.e.; PI3K, MEK or NFKB), or the mechanism of the apoptosis itself (i.e.; Bcl2, Bclx, Apaf1, etc.).
Epigenetic alterations, although not structural, provide a source of large transcriptomic variations. Therefore, the identification of these alterations in tumours may provide a suitable footprint for making clinical decisions.
Whatever the alteration, it provides the basis for variation and therefore for differential prognosis and/or treatment response. Whole genome analysis to identify significant variations coupled to screening programmes tries to identify and validate significant markers. However, recent results from the analysis of the primary tumours and its metastasis showed a high rate of variation, which was also observed between different sites of the same primary tumour, showing an unexpected high degree of clonal evolution in most tumours5-8. This variability makes it difficult in the identification of efficacious biomarkers since each may have its own part to play and in some cases may be even contradictory. The application of targeted therapies may help by combining different treatments according to the driver mutation found. But clearly, much more research in this area needs to be done.
Transcriptomic markers
Transcription is the result of genetic or epigenetic alterations that control mRNA synthesis. However, it is also the result of miRNA expression and, commonly overlooked, of the mRNA degradation machinery. Therefore, transcription profiles result from the integration of many other processes and might, in principle, be of use for more general biomarkers9. The improvement of microarrays and transcriptomic studies led to the identification of a set of genes whose behaviour helped to predict such response, regardless of the specific mutation each tumour may carry. These genes reported in transcriptome profiles are far from being causal (or driver) genes, but contribute to identify suitable patients for a treatment10.
The current trend for the use of these markers is by quantitative PCR (Q-PCR) either by single gene or in cards applied to a set of genes at the same time. One difficulty of these applications is the needed robustness of each transcriptomic biomarker found. Since different PCR machines can give slightly different results, the application of a decision tree based on quantitative measurements need to be exhaustively validated in different laboratories. Presumably, the expression levels in non-tumoral tissue might be useful as a point of reference among different laboratories. Furthermore, profiles composed of many genes need a clear and logical algorithm that evaluates not only individual levels of each gene but also some clinicopathological features of the patient to provide a clear response.
Currently, some of these profiles are used in oncology. In breast cancer, there are currently two genomic tests; MammaPrint and Oncotype DX. They analyse the profiles of 70 or 21 genes respectively, but their patient spectrum of application is very limited11-13. Despite their drawbacks, these tests have served as an inspiration to many scientists to provide profiles for many tumour types and treatments.
microRNAs or miRNAs, the small fragments of RNA that regulate the mRNAs in a target specific fashion, have been a matter of recent interest. miRNAs profiles, as a reflection of transcriptome variations and also able to provide causal alterations on genes, are being regarded as suitable markers for treatment14. Many studies report one or more miRNAs responsible for the response to treatments, many of them applied to cytotoxics15. However, it is still early for these markers to be of broad use in clinical decisions.
Proteomic markers
The phenotype of a cell is determined by proteins and cannot be predicted by genomics alone. Indeed, protein expression levels are poorly correlated to messenger RNA expression levels16,17, and post-translational modifications such as phosphorylation, glycosylation and proteolytic processing, which are common events, have the potential to significantly modify protein functions and characteristics of the cell or tissue where the protein is expressed18. Recent progress in clinical proteomics is mostly contributed by sophisticated new methodologies for proteome analyses. Different proteomics tools such as two-dimensional polyacrylamide gel electrophoresis (2DPAGE, DIGE), protein arrays, isotope coded affinity tags (ICAT), iTRAQ and SILAC, are approaches being implemented in cancer research to identify proteomic portraits as a complement to histopathology to facilitate individualised selection of therapeutic combinations. Less well-developed techniques rely on highly specialised technology that need an extra step of technical adaptation to be commonly used in most hospitals. Protein biomarkers need to be adapted to immunohistochemistry, ELISA, western blot or other techniques easy to handle in most hospitals without a top proteomic facility.
Metabolomic markers
As a result of enzymatic activity, the chemical environment of the cells changes between normal and tumour cells and in the same regard, presumable changes between responders and non-responders cells and tumours. Looking for these changes in biochemical compounds is also a topic of interest in the coming decades. However, the complexity of the techniques (i.e.; NMR) and the difficulty to apply to common clinical use hampers its true application. Many compounds have been identified, but further validation in independent cohorts and by a different (easily handled) method is lacking in the continuation of many works.
Not there yet
Despite the large amount of studies, there are very few truly validated biomarkers. Most biomarkers fail at clinical application. To improve the quality of publications on biomarkers, the Strategy Group of the Program for the Assessment of Clinical Cancer Tests (PACCT) and a working group of an NCI-EORTC collaboration developed the guidelines for the information that should be included in all publications about tumour markers, the REMARK criteria, REporting recommendations for tumour MARKer prognostic studies (http://cdp.cancer.gov/scientificPrograms/pacct/remark.htm). The recommendations appear in the web, were published in many journals and should be of compulsory consultation.
In many cases, as briefly mentioned before, the complex technology used to identify biomarkers is difficult to reproduce in common clinical practice. In these cases, an adaptation of the technique is necessary to allow easy and non-expert handling of the technology.
The origin of the samples from what to extract the biomarker is also matter of debate. Ideally, tumour sample and nearby (same tissue of origin) non-tumoral control from the same patient (matched samples) are the ideal samples to identify biomarkers since they are the true tissue matter of disease, and the biomarkers are expected to be contained. However, in many cases this is impossible due to the difficulty in acceding to the tumour mass or the small amount of sample recovered. Some biopsies are performed with small bits which will only provide enough material for anatomopathological diagnosis. And if there is large clonal variation in the tumour, will a small biopsy have any relevance in clinical decisions? Moreover, many tumours (such as some late stage tumours in which biopsies will not provide any further benefit to the patient) will be difficult to find ethical arguments to extract samples by surgery even to perform the studies. It is always better to perform a non-invasive procedure than an invasive one. In this regard, surrogated tissues can supply a good tissue sample. Blood, lipid tissue, saliva, etc. are always better perceived for biomarker studies. However, do the biomarkers found in these surrogated tissues correlate to the tumour? Some direct comparison between tissues of the same patient showed that only a small fraction of alterations found in the tumour are found in other surrogated tissues19. Although it is possible to find some valuable markers, we may be losing many of the most significant ones and, furthermore, an additional validation step will be needed.
Conclusions
Personalised medicine talks about how to identify the best treatment for each patient20. Biomarkers are the response. Either in the form of genomic alterations, transcription, proteomic or metabolomic profiles, the identification of biomolecules able to predict the response to any given drug is the key to tailored treatment. Current whole genome sequencing projects will evolve to identify targets suitable to be attacked by new drugs (targets will become biomarkers then), either alone or, more probably, in combinations. However, the tumours are ‘living creatures’ subject to ‘Darwin’s law’. And, very likely, many tumours treated will survive like species adapting to new environment. And here we go again, new analysis, new markers and new treatments.
Acknowledgments
This work was supported by grants from Fondo de Investigación Sanitaria (PI12/00137), RTICC (R12/0036/0028) and Consejeria de Ciencia e Innovacion of the Junta de Andalucia (CTS-6844). AC’s laboratory is also funded by a fellowship from Fundacion Oncologica FERO.
Biography
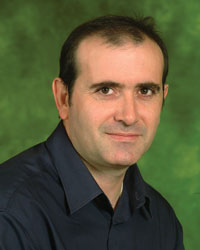
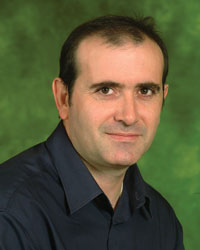
Amancio Carnero, Instituto de Biomedicina de Sevilla, Consejo Superior de Investigaciones Científicas
Amancio Carnero received his PhD in Molecular Biology from the Univesidad Autónoma de Madrid, Spain. He moved to CSHL (USA) and later to London (ICH and WIBR) where his research interests focused on the cell cycle, replicative senescence and cellular immortalisation. In 2001, he returned to Spain to lead a group at the CNIO (Madrid). At the end of 2009, Amancio moved to Sevilla to lead the Molecular Biology of Cancer Lab at the IBIS. The current research in Amancio’s lab focuses on the identification of new genes involved in cancer, its characterisation and translation to clinic, and the identification and validation of new targets for anticancer drug discovery.
References
- Estevez-Garcia P, Castano A, Martin AC, Lopez-Rios F, Iglesias J, Munoz-Galvan S, Lopez-Calderero I, Molina-Pinelo S, Pastor MD, Carnero A, Paz-Ares L, Garcia-Carbonero R. PDGFRalpha/beta and VEGFR2 polymorphisms in colorectal cancer: incidence and implications in clinical outcome. BMC Cancer 2012;12:514
- Broxterman HJ, Gotink KJ, Verheul HM. Understanding the causes of multidrug resistance in cancer: a comparison of doxorubicin and sunitinib. Drug Resist Updat 2009;12(4-5):114-126
- Buac D, Shen M, Schmitt S, Kona FR, Deshmukh R, Zhang Z, Neslund-Dudas C, Mitra B, Dou QP. From Bortezomib to other Inhibitors of the Proteasome and Beyond. Curr Pharm Des 2013;19(22):4025-4038
- Galluzzi L, Senovilla L, Vitale I, Michels J, Martins I, Kepp O, Castedo M, Kroemer G. Molecular mechanisms of cisplatin resistance. Oncogene 2012;31(15):1869-1883
- Pavelka N, Rancati G, Li R. Dr Jekyll and Mr Hyde: role of aneuploidy in cellular adaptation and cancer. Curr Opin Cell Biol 2010;22(6):809-815
- Polyak K. Heterogeneity in breast cancer. J Clin Invest 2011;121(10):3786-3788.
- Zhang L, Znoyko I, Costa LJ, Conlin LK, Daber RD, Self SE, Wolff DJ. Clonal diversity analysis using SNP microarray: a new prognostic tool for chronic lymphocytic leukemia. Cancer Genet 2011;204(12):654-665
- Landau DA, Carter SL, Stojanov P, McKenna A, Stevenson K, Lawrence MS, Sougnez C, Stewart C, Sivachenko A, Wang L, Wan Y, Zhang W, Shukla SA, Vartanov A, Fernandes SM, Saksena G, Cibulskis K, Tesar B, Gabriel S, Hacohen N, Meyerson M, Lander ES, Neuberg D, Brown JR, Getz G, Wu CJ. Evolution and impact of subclonal mutations in chronic lymphocytic leukemia. Cell 2013;152(4):714-726
- Li X, Quigg RJ, Zhou J, Gu W, Nagesh Rao P, Reed EF. Clinical utility of microarrays: current status, existing challenges and future outlook. Curr Genomics 2008;9(7):466-474
- Hyams DM. Understanding tumour profiling and assessing treatment. Manag Care 2008;17(7 Suppl 7):4-8; discussion 17-18
- Ross JS. Multigene classifiers, prognostic factors, and predictors of breast cancer clinical outcome. Adv Anat Pathol 2009;16(4):204-215
- Mook S, Van’t Veer LJ, Rutgers EJ, Piccart-Gebhart MJ, Cardoso F. Individualization of therapy using Mammaprint: from development to the MINDACT Trial. Cancer Genomics Proteomics 2007;4(3):147-155
- Arpino G, Generali D, Sapino A, Lucia del M, Frassoldati A, de Laurentis M, Paolo P, Mustacchi G, Cazzaniga M, De Placido S, Conte P, Cappelletti M, Zanoni V, Antonelli A, Martinotti M, Puglisi F, Berruti A, Bottini A, Dogliotti L. Gene expression profiling in breast cancer: a clinical perspective. Breast 2013;22(2):109-120
- Healy NA, Heneghan HM, Miller N, Osborne CK, Schiff R, Kerin MJ. Systemic mirnas as potential biomarkers for malignancy. Int J Cancer 2012;131(10):2215-2222
- Cho WC. Great potential of miRNAs as predictive and prognostic markers for cancer. Expert Rev Mol Diagn 2012;12(4):315-318
- Honda K, Ono M, Shitashige M, Masuda M, Kamita M, Miura N, Yamada T. Proteomic approaches to the discovery of cancer biomarkers for early detection and personalized medicine. Jpn J Clin Oncol 2013;43(2):103-109
- Hung KE, Yu KH. Proteomic approaches to cancer biomarkers. Gastroenterology 2010;138(1):46-51 e41
- Pastor MD, Nogal A, Molina-Pinelo S, Carnero A, Paz-Ares L. Proteomic biomarkers in lung cancer. Clin Transl Oncol 2013
- Molina-Pinelo S, Suarez R, Pastor MD, Nogal A, Marquez-Martin E, Martin-Juan J, Carnero A, Paz-Ares L. Association between the miRNA signatures in plasma and bronchoalveolar fluid in respiratory pathologies. Dis Markers 2012;32(4):221-230.
- Mehta R, Jain RK, Badve S. Personalized medicine: the road ahead. Clin Breast Cancer 2011;11(1):20-26