Raman spectroscopy as a blood glucose monitoring tool
Posted: 30 June 2017 | Vinay Bhardwaj (Rutgers University), Vinod Gaur (University of Washington) | 1 comment
European Pharmaceutical Review, Issue 2 of 2016 focused on nanotechnology and stem cell technology to monitor glucose and prevent diabetes, respectively. The aim of this review is to sensitise the technology innovators in this field by providing expert opinions on biomedical products and technologies that have come the closest so far to fulfilling the requirements of a successful diabetes monitoring product…
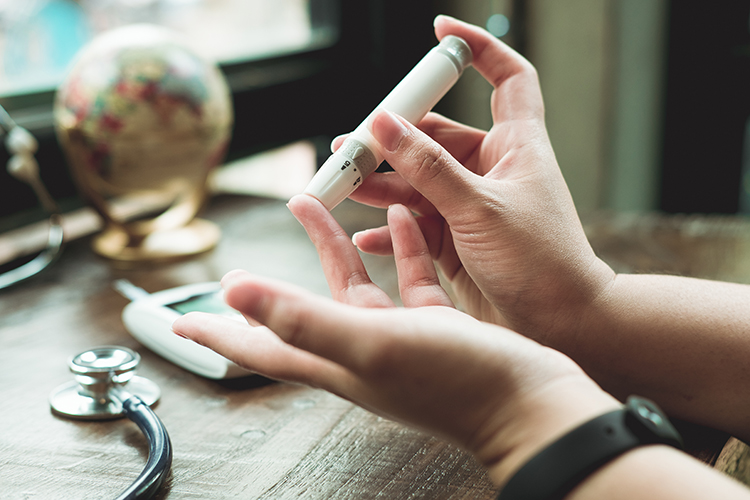
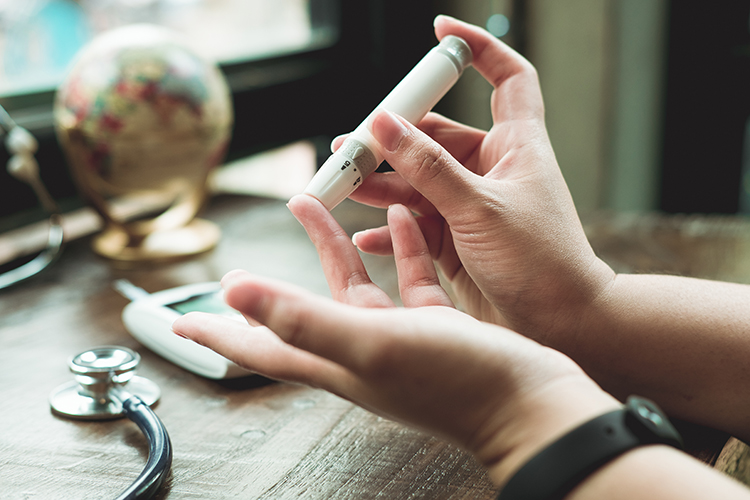
Figure 1: First-generation glucose test strips and glucometers launched in 1965 by (left) Boehringer Mannheim, now Roche Diagnostics, and (right) Miles-Ames Medical Lab, now Bayer AG Germany. The test was based on a simple reaction between copper and sugar to produce a colour change that was proportional to glucose concentration, and the glucometers were based on reflectance.
These include being accurate, painless, affordable, in palm-of-public for in-home use, real-time and capable of allowing on-demand delivery of insulin in response to rising glucose levels. The evolution of glucose sensor technologies and their technical analysis in the context of the degree of error, pain, portability, cost and sampling process will be summarised, with a focus on glucose sensors based on principles of reflectance, electrochemical reaction, and light scattering Raman spectroscopy.
According to the International Diabetes Federation, 415 million people worldwide have diabetes, and this is expected to increase by 55% by 2040.^1 Although 12% of total global health expenditure, or $673bn, is spent on diabetes, 5 million people still die from the disease each year.
Jorge Rees, a physician at Guy’s hospital in London set the platform for the glucose sensor market in 1838 by identifying a direct association between diabetes and excess sugar in blood plasma. Over the course of the next century, significant progress was made in designing chemical reactions to detect glucose. The reaction of copper salt with sugar at high temperature was the principle reaction behind glucose detection. But it wasn’t until 1945 that the first glucose test kit – Clinitest from Dr Miles medical company in the US – hit the market. However, Clinitest and any other glucose kit using patients’ urine as their sample are unsatisfactory and dangerous.^2 Unlike urine, which is the excretory pathway, blood is the first and primary site of glucose transport after its catabolic generation from food that we ingest. Scientifically, glucose analysis in the blood is the correct sampling process to measure clinically accurate levels of body glucose. Therefore, measurement of glucose in blood became the standard medical practice and is still the gold-standard reference sampling method when developing any new glucose sensor technology for diabetes monitoring. Dextrostix (Miles-Ames lab) and ChemStrip bG (Boehringer Mannheim) were the most successful, widely-used blood glucose test strips, but they were only semi-quantitative, and were restricted to clinics and hospitals for use by physicians only after prescription.
SMBG glucometers
The concept of routine self-monitoring of blood glucose (SMBG) has been standard medical care since it was first introduced in the 1970s for diabetes self-management. SMBG disrupted the market by introducing sensor systems that were affordable and simple to use by diabetic patients without any need to visit a doctor. Boehringer Mannheim, Miles-Ames lab, Kyoto Daiichi, and LifeScan were the main manufacturers to commercialise glucose meters for SMBG, all of which were reflectance meters (Figure 1).
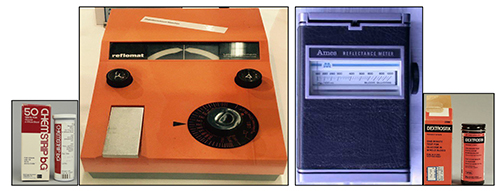
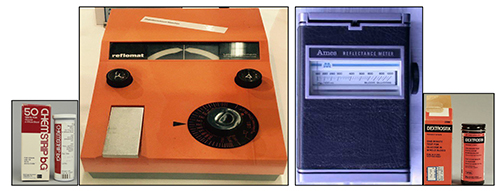
Figure 1: First-generation glucose test strips and glucometers launched in 1965 by (left) Boehringer Mannheim, now Roche Diagnostics, and (right) Miles-Ames Medical Lab, now Bayer AG Germany. The test was based on a simple reaction between copper and sugar to produce a colour change that was proportional to glucose concentration, and the glucometers were based on reflectance.^3
LifeScan’s OneTouch glucometer can be considered second generation, as it was simple to use and carry for self-monitoring. Results were generated within 45 seconds of putting a small volume of blood on a test strip already inserted in the meter. There was no need to wash and wipe the strip, so it was truly a one-touch measurement. Accuracy was still an issue, however, with around 30% deviation from laboratory testing.^4 A whole family of OneTouch glucometers are now available with improved performance and accuracy.
The American Diabetes Association (ADA) regularly revises and releases standards of medical care in diabetes for patients and clinicians.^5 In 1987 ADA revised the medical guidelines for SMBG, lowering the glucometer deviation with respect to reference laboratory tests to 15%.^6 Clark and Lyons’ electrochemical technique, first introduced in 1962 at Cincinnati Children’s Hospital in USA,^7 and Clarke’s error grid analysis in 1987,^8 became popular technologies to detect and medically analyse glucose data.
Two salient features of the electrochemical technology are, first, the use of glucose oxidase enzyme to specifically metabolise glucose and, second, the by-product of this enzyme-specific reaction is electrochemically active and generates a current. Therefore, the current intensity is directly related to glucose concentration and is measured using an inexpensive amperometer. Over the time, this electrochemical sensor technology was refined^9 to completely replace reflectometers. ExacTech by MediSense – marketed in 1987 and developed by the universities of Oxford and Cranford – was the first such electrochemical or electrode-based glucose sensor.
Invention to acquisition
Glucometers based on reflectance and electrochemical principles became widely accepted, but the electrochemical glucose sensor became more dominant owing to its affordability, simplicity, portability and accuracy – pre-requisites for SMBG by diabetic patients. The key players, or ‘inventors’, of these two technologies were Boehringer Mannheim in Germany and Ames lab in the US for reflectometers, and LifeScan in the US for electrochemical technologies. The growing market post-1990s changed the focus from innovation to acquisition. All these innovator companies were finally acquired: Ames by Bayer in 1997; Boehringer Mannheim by Roche Diagnostics in 1998; MediSense and TheraSense by Abbott in 1987 and 2004; LifeScan by Johnson & Johnson in 2006; plus a few other small companies (Figure 2).
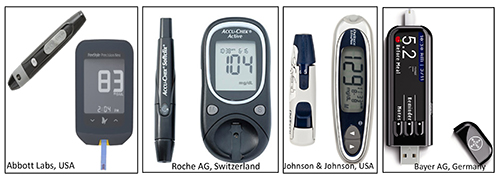
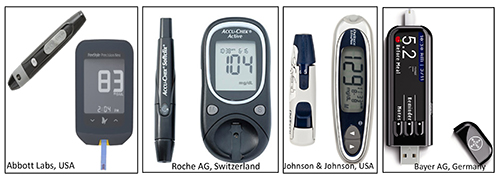
Figure 2: Some of the leading global companies who acquired electrochemical glucose sensor technologies from the early inventors.
Progress in CGMS
To manage diabetes, ADA recommends the regular self-monitoring of blood glucose: four times daily for type 1 diabetes patients; twice daily for type 2; and six to ten times, or even more, per day for patients on an intensive insulin regimen.5 Currently, most patients and clinicians use a painful finger-prick sampling procedure and glucose oxidase-based electrochemical glucometers for self-monitoring of blood glucose. Compare finger-pricking several times daily and self-administering insulin injections with a smart biomedical device that sits around the belly to continuously manage glucose levels through a feedback control/algorithm between glucose sensor and insulin injector. However, Dexcom’s continuous glucose monitoring sensor/receiver (Figure 3), has recently been recalled by the US-FDA under a class I recall. This is the most serious recall, suggesting that the device may result in serious injuries or death. Since Dexcom obtained FDA approval in 2006 for its CGMS technology, it has introduced a series of devices that have been able to corner some of the narrow niche market.
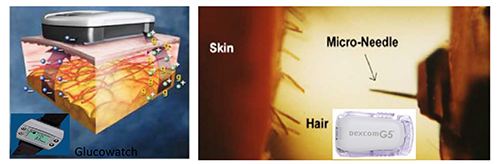
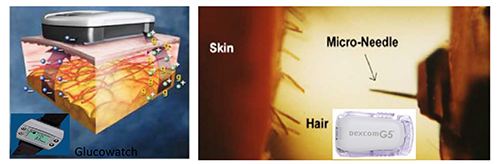
Figure 3: Dexcom and Freestyle (left) and GlucoWatch (right) have core sensor technology similar to electrochemical glucose sensing, except the sampling process, transdermal needle insertion in FreeStyle and Dexcom, and reverse iontophoresis in GlucoWatch to allow flow of interstitial glucose into the sensor chamber which has glucose oxidase enzyme.
Another example based on a similar sampling procedure (transdermal insertion of needle to continuously monitor glucose in interstitial fluid) is FreeStyle from Abbott Labs (Figure 3, left). FreeStyle is less invasive than Dexcom, has a longer shelf-life and a thinner needle.10 Some of the later startup US companies who terminated their efforts to build CGM sensor technologies based on the transdermal insertion of a needle are Integra in Minneapolis, Spectrx in Georgia, Kumetrix in California, and several others.
The reverse iontophoresis sampling process behind GlucoWatch is another example of FDA-approved CGMS (Figure 3, right). However, Cygnus is no longer manufacturing these watches because the technology was neither user-friendly, nor clinically valuable, for the following reasons:
- It required a three-hour warm-up period
- It could not replace, but only supplement, the traditional fingerpick technology
- Poor accuracy, leading to false alarms and misguided management
- It was painful, as the sampling process relied on electrical modulation of the skin to release glucose.
Non-invasive CGMS
Unlike invasive fingerpick and minimally-invasive microneedle, or stimulated-release sampling followed by electrochemical detection, the use of light ‘spectroscopy’ is scientifically the best way to build a completely non-invasive system to monitor glucose in situ. Efforts to use label-based fluorescence spectroscopy,11 label-free radio wave impedance spectroscopy,12 mid-infrared absorption spectroscopy,13 near-infrared Raman spectroscopy,14 and a few others, have been made. However, there is no non-invasive CGM sensor technology on the market yet. Pendra, from Pendragon Medical in Zurich, developed a completely non-invasive technology based on impedance spectroscopy in 2003. However, the company terminated the technology development due to capital constraints and the poor technical design. Although the technology was miniaturised to fit inside a wristwatch, it was a failure because it was based on ‘indirect detection’ of glucose, based on change in impedance of the skin with different levels of glucose. Therefore, their device had poor correlation in accuracy when compared to clinical-standard finger prick levels.
Consumers’ decisions are primarily driven by affordability and ergonomics, such as integration of the technology to their watches, and most of the CGM sensor technologies discussed until now have fulfilled these requirements. However, clinically, the requirement is for a high degree of accuracy with ≤ 5% error when compared to the gold-standard reference tests, and ADA’s new accuracy limits were set in 1996.^15 Metabolically, there will be some lag in the transport of glucose from blood to interstitial fluid, which can lead to some differences in the readings of intestinal glucose vs blood glucose.
Raman spectroscopy, just like FTIR and NIR, is a type of molecular spectroscopy that provides direct chemical signatures of the glucose molecules that make molecular spectroscopy a promising tool to develop non-invasive CGM sensor technologies. However, glucose has a very small cross-sectional area, and hence its detection through skin using molecular spectroscopy is challenging. The discrimination of glucose from its isomers further complicates the detection process. Compared to any other molecular spectroscopy, Raman gives rich information about the sample, allowing glucose-specific detection and discrimination from isomers and background that also has biomarkers of skin and the blood (Figure 4).
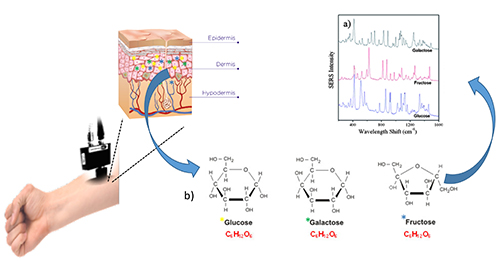
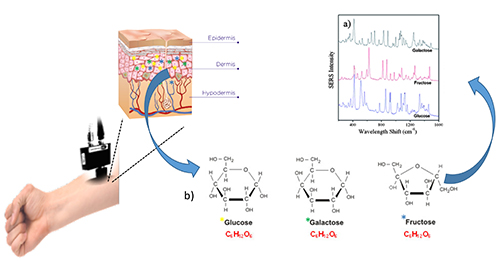
Figure 4: Non-invasive Raman spectroscopy for continuous and pain-free glucose monitoring. The schematic show that NIR laser can be shined on forearm to detect and discriminate glucose from its isomers like galactose and fructose based on their molecule-specific Raman light scattering or shift.
The process of incorporation of internal standard biomarkers can both address and compensate for the likely chances of error due to collagen content with respect to age of the patient, and melanin content with respect to race or skin colour, etc. No doubt, compared to light absorption-based FTIR and NIR, Raman spectroscopy is a weak phenomenon because it is based on light scattering. However, custom-developed algorithms or buying a chemometrics package to enumerate accurate signatures or peaks responding to glucose levels, can easily overcome this limitation.
A few of the tips and tricks that should be considered when developing a Raman sensing system for non-invasive CGMS are as follows:
- Configure highly sensitive yet safe optics: a combination of all suitable optics should be tested, such as the 785 vs 820 nm laser, different gratings and slit sizes, and the detector efficient in glucose or biochemical and biological range.
- Focus on system configuration using real-world subjects, because relying on Raman active standards and in vitro analysis can sometimes be distracting and misleading. For example, lower gratings might give intense signals but can miss glucose specific information when testing in vivo.
- Do not rely on manual or molecular analysis of peaks, but instead take a parallel approach using molecular as well as a machine-learning approach to finding multiple peaks. The area under the peak is a preferable approach to peak intensity, as biological samples have a strong background.
- Include internal standard biomarkers specific to skin in order to normalise the readings with respect to skin colour, age and body vitals. Many technologies and the startup industries in this business fail because they are unable to develop a robust CGM sensor technology and a process that can cover an entire range of diabetic patients, hypoglycemic to hyperglycemic (50-350 and even higher mg/dL).
Future directions
Accuracy, affordability and portability are the three major drivers that determine the success of sensor technology. There is no doubt that electrochemical glucose sensor technology will continue to be most accurate, affordable and suitable for in-home use by patient for self-monitoring and management of diabetes. However, the pain, the biohazard regulation and the likely chances of infectious disease transmission through blood are some of the major concerns of patients and clinicians that push innovators to develop technologies that are pain-free and do not involve direct contact with blood (Figure 5).
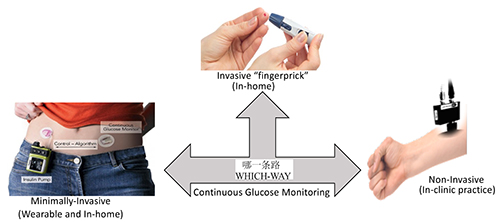
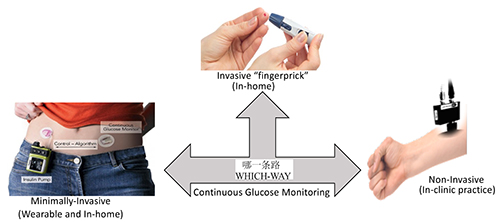
Figure 5: The schematics highlight progress and future of the glucose sensor technology. Although significant progress has been made in engineering painless technologies for continuous glucose monitoring, the fingerpick is still the FDA-approved and ADA-recommended gold-standard technology available to patients for self-monitoring and self-managing diabetes.
Devices with minimally invasive wearable technologies, which are usually based on skin modulation or transdermal puncture by needle to release glucose for facile detection, have shown promising results. However, the poor accuracy and required continuous calibration with respect to the gold-standard finger prick process has restricted their use as a technology to supplement and not replace the finger prick procedure. Molecular spectroscopy, in particular Raman spectroscopy, has the capability of direct in situ detection of glucose with high specificity and accuracy. Additionally, there has been great success in miniaturisation of Raman spectrometers to handheld designs of around one kilogram, with more than two-dozen handheld products in the US market alone. However, the cost associated with the instrument might restrict its use to clinical settings, at least until Raman optics can be integrated to the regular vital meters such as those for testing blood pressure. Unlike all other technologies discussed in this review, Raman spectroscopy will not require any additional medical products like blood lancets or reaction chambers.
Biography
References
- International Diabetes Federation. About diabetes, facts and figures 2015 to 2040. Available at: idf.org/about-diabetes/what-is-diabetes.
- Angaran DM, Smith DS, Birnbaum M. The use of urine test for indirect estimation of blood glucose levels in the critically ill. American Journal of Hospital Pharmacy.1980;37:950-956.
- Clarke SF, Foster JR. A history of blood glucose meters and their role in self-monitoring of diabetes mellitus. British Journal of Biomedical Science. 2012;69(2):83-93.
- Leroux ML, Desjardine PRE. Ward level evaluation of the ‘OneTouch’ glucose meter. Clinical Chemistry. 1988;34(9):1928.
- American Diabetes Association. Standards of medical care in diabetes-2017. Diabetes Care. 2017;40(1):S1-S135.
- American Diabetes Association. Consensus statement on self-monitoring of blood glucose. Diabetes care. 1987;10(1):95-99.
- Clark LC, Lyons C. Electrode systems for continuous monitoring in cardiovascular surgery. Annals of the New York Academy of Sciences. 1962;102(1):29-45.
- Clarke WL, Cox D, Frederick LAG et al. Evaluating clinical accuracy of systems for self-monitoring of blood glucose. Diabetes care 1987;10(5):622-628.
- Wang J. Electrochemical Glucose biosensors. Chemical Review. 2008;108:814-825.
- Chen C, Zhao XL, Li ZH, et al. Current and emerging technology for continuous glucose monitoring. Sensors. 2017;17(182):1-19.
- Klonoff DC. Overview of fluorescence glucose sensing: A technology with a bright future. Journal of Diabetes Science and technology. 2012;6(6):1242-1250.
- Talary MS, Dewarrat F, Huber D, et al. Noninvasive impedance-based continuous glucose monitoring systems. IFMBE Proceedings Berlin. 2007;17:636-639.
- Liakat S, Bors KA, Xu L, et al. Noninvasive in vivo glucose sensing on human subjects using mid-infrared light. Biomedical Optics Express. 2014;5(7):2397-2401.
- Pandey R, Paidi SK, Valdez TA, et al. Noninvasive monitoring of blood glucose with Raman spectroscopy. Accounts of Chemical Research. 2017;50:264-272.
- American Diabetes Association. Clinical practice recommendation. Diabetes care. 1996;19(1):S62-66.
Issue
Related topics
Analytical techniques, Biomarkers, Nanoparticles, Raman Spectroscopy, Stem Cells, Technology
The growing prevalence of diabetes and other infectious diseases around the globe is expected to drive the growth of the market during the forecast period.