Revolutionising Raman with the transmission technique
Posted: 3 July 2015 |
All regulatory agencies require that manufacturers accurately determine the amount of drug in a medicinal product so that the product can be deemed fit for patients. This forms part of batch release testing performed by Quality Control (QC) laboratories. The drug assay and/or content uniformity of the dosage units is typically performed by a wet chemistry technique that has not significantly changed in the industry for four decades.
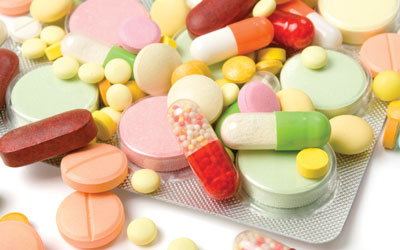
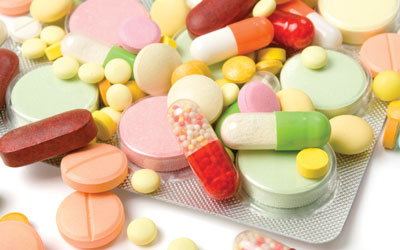
High performance liquid chromatography (HPLC) is widely used by laboratories for that purpose, and automated sample preparation systems are available. But there are drawbacks to these methods. This article explores how Transmission Raman now has the potential to revolutionise the release testing of pharmaceutical product in a QC laboratory.
HPLC is a slow and labour-intensive technique as analyses require the preparation of samples and standards, as well as instrument run times measured in hours. In addition, the sample preparation is typically conducted by bench chemists and human error can result in inaccurate results, leading to lengthy investigations and delays in product release. Meanwhile, for products that are infrequently tested, the cost of using automated sample preparation systems can be prohibitive. Ultra performance liquid chromatography (UPLC) is another method used – it does reduce the duration of the runs on the instrument, but the amount of time spent by laboratory personnel on sample preparation remains unchanged.
Alternative approaches
Actavis UK Ltd undertook a project to modernise how samples were tested in the QC laboratory, making significant improvements to the supply chain and speeding up the process of product supply to the market. After reviewing the technologies that can non-destructively test solid oral medicinal products, Transmission Raman spectroscopy was identified as the most chemically specific alternative that avoids sub sampling issues from the tablets or capsules. The technique eliminates sample and standard preparation time, with routine sample analysis in just minutes, and as the added benefit of eliminating environmental waste. Training to perform the routine analysis is extremely quick compared to the conventional chromatography techniques.
The outcome of this project is that Actavis has developed and validated replacement QC release test methods using Transmission Raman spectroscopy for the quantitation of active pharmaceutical ingredients (APIs) in intact tablets (bulk assay and Uniformity of Content). The non-destructive Raman methods also perform the identification of the API in the tablets, thereby fulfilling the identification requirement for batch release. Standards are not required at the time of analysis by QC personnel, resulting in very short turn-around times for batch release tests.
The Transmission Raman methods have received regulatory approval by the UK Medicines and Healthcare Products Regulatory Agency as alternative methods for the release of commercial product to the market. Prior to this, HPLC and/or thin layer chromatography were the licensed methods for the bulk assay, uniformity of content and ID testing.
Equipment – Advantages of Transmission Raman over conventional Raman spectroscopy
The equipment used for the Raman method and its operation were described in the variation. Transmission Raman spectroscopy is a variant of traditional Raman spectroscopy: in traditional backscattered Raman spectroscopy, monochromatic light from a laser is focused onto a small area at the surface of a sample, and the scattered light is collected on the same side as the laser excitation. Due to the small sampled volume, traditional Raman spectroscopy is very prone to sub-sampling: the drug content near the surface of the sample illuminated by the laser in not representative of the drug content in the whole sample. Sample spinning or wide-illumination optics can increase the sampled area, but even then sampling is limited to the sample’s surface, resulting in a small sampled volume.
In Transmission Raman spectroscopy, the laser light illuminates a wide area on the whole intact sample, and the light is collected on the opposite side of the sample: the laser travels through the sample, generating Raman signal from the entire cross section of the sample. As a result, the Raman signal is made up of the contribution from throughout the sample, and is representative of the whole tablet.
The Raman method was developed and validated on Cobalt Light Systems’ Transmission Raman System TRS100. The TRS100 uses an 830nm laser, delivering up to 0.65 Watt of laser power at the sample; the laser spot size is adjustable (up to 8mm wide) and the collection optics can be selected to accommodate different sample sizes. For the methods approved by the regulator, the laser and collection optics sizes were set such that they match the size of the tablets.
Regulatory framework and approval
Raman spectroscopy is a relatively recent technique, and does not have dedicated regulatory guidelines for variations. It is, however, similar to near infrared spectroscopy, since both methods:
- require the collection of spectral libraries for initial calibration (but not for routine analysis)
- rely on chemometrics to relate the spectra to the sample composition
- follow a method life cycle approach, to ensure the method remain in a validated state.
The validation of the Raman method therefore followed the general ICH guidelines for method validation[1], as well as the guidelines specific to NIRS applications[2], including the description of the method life cycle’s management.
Due to the innovative technology used and the expected long regulatory review, the submissions to add Raman methods as an alternative to the already-licensed tests methods (HPLC, TLC) were submitted as type II variations, even though they were for the replacement or addition of test procedure for finished product[3]. As regulatory reviews become more frequent and faster, these variations are likely to become type IB.
Methodology
Principles of chemometrics
As part of the submission, the basics principles of chemometrics were described, with supporting figures to help with the assessment of the methods: each Raman spectrum records the intensity of the light at multiple wavelengths, and is therefore composed of several hundred data points. For each of the many wavelengths, the Raman spectrum has a contribution from the chemical constituents of the sample. Frequently, for the same wavelength, several constituents contribute to the sample’s overall spectrum due to overlapping Raman bands (see Figure 1).
It is therefore not possible to establish, as for HPLC, a simple univariate relationship between peak area (or peak height) and concentration of the API. Instead, chemometrics are used to establish the multivariate relationship between the Raman spectrum of whole tablets, and API concentration (spectra from pure API and/or pure excipients are not needed).
Chemometrics requires spectral pre-processing to minimise spectral variation that is not related to the API concentration (e.g., baseline correction, normalisation), and to maximise the spectral variation that does relate to the API. Chemometrics then correlate pre-processed spectra, collected non-destructively from calibration tablets, with reference API concentration values generated by a reference method for those same tablets (e.g., the destructive HPLC batch release method). Once established, the resulting relationship, or ‘chemometric model’, is re-used to determine the API concentration in new spectra, from new tablets (see Figure 2).
To validate the Raman chemometric model, new samples from batches not used during the calibration were analysed by both Raman and HPLC. The agreement between both method’s results were compared and had to meet pre-determined acceptance criteria. In addition, the Raman method met the traditional ICH requirements (linearity, precision, accuracy etc.).
The regulatory submission also described how, as a pre-requisite to reporting a result, the chemometric model also performs a spectral check (Hotelling T2 and Q Residuals) to verify that a sample’s spectrum complies with the expected type of sample, and is within the method’s design space defined by the calibration samples (ingredients present in the sample, ranges of API and excipients concentrations). Tablets made from blends deliberately outside of the model’s design space (spiked with an incorrect excipient, or incorrect excipient moisture, or placebo) were tested to show that the model will detect and reject out-of-scope samples.
Calibration and validation blends
The Raman methods were developed for direct compression single-strength products with 5mg of API (ranging from c.a. 5% and to c.a. 10% w/w of API in the formulations, respectively); the APIs were different for the products.
In order to calibrate the Raman method, designed experiments were conducted to produce blends with ranges of API concentrations ranging at 70%, 85%, 115% and 130% of nominal, on a lab scale (c.a. 75 grams each). Variation in raw material lots, in the excipients’ concentrations relative to the API, and in the excipients’ concentrations relative to each other was also included in those calibration blends, to make the calibration robust.
Each of the blends was then compressed on a lab-scale compression machine to make compacts with the same weight and diameter as production scale samples. Samples from production batches (c.a. 100% nominal) were also used for the calibration.
For each product, compacts were also prepared for four lab-scale validation blends, with API concentrations of 70%, 85%, 115% and 130% nominal, as well as variation in excipients relative to the API and relative to each other (to delimit the method’s design space). The formulations of the validation blends were different from the calibration blends, to ensure they were independent of the calibration. Samples from different production batches were also used for the validation.
For each product, two lab-scale blends with deliberately incorrect formulations, and a lab scale placebo blend, were also prepared to validate that the chemometric model’s spectral check would reject samples that were compositionally incorrect or outside of the method’s design space.
Feasibility study/method conditions
Prior to starting the collection of the Raman data for the method calibration and validation, a short feasibility study was carried out to determine the acquisition time required to achieve a certain precision, the settings for the laser spot size and collection optics’ size, and to determine the laser power at which sample heating damage would occur.
As possible sample damage is a known issue in traditional Raman spectroscopy and is mentioned in the pharmacopoeia, this risk was specifically addressed during the method feasibility. No sample damage was observed at the TRS100’s maximum power: since the TRS100’s laser spot is several millimetres wide, the laser power was spread over a wide area (contrary to traditional Raman, where the laser power is focused on an area several orders of magnitude smaller) and sample damage induced by localised heating did not occur.
Results
To demonstrate that the proposed Raman methods were equivalent to the already-licensed methods and could be used for batch-release testing, the results of the method validation were presented in the submission.
In addition to the typical ICH method validation acceptance criteria, the validation included:
- the calculation of the standard error of prediction and the bias of the Raman method, as required in the NIR spectroscopy guidelines
- the comparison of the variance of the results by HPLC and Raman, since the method is intended for uniformity of content testing
- the description of the chemometric model’s latent variables and how they relate to the composition of the sample
- the distribution of the percentage relative standard deviation for (non-destructive) repeat measurements on individual tablets by the Raman method.
In the variation, the results were provided both in concentration of API in the formulation (% w/w), which the Raman chemometric model measures, and in amount of API per tablet (calculated from the API% w/w and the tablet weight).
Individual tablets results determined by the Raman method were plotted as a function of the matching results determined by the HPLC method (Figure 4). For bulk assay, the mean of 10 individual tablets’ results determined by Raman was plotted as a function of the one matching HPLC result, for the different populations.
The results obtained demonstrated that Transmission Raman was a very accurate technique, equivalent to HPLC, for QC release testing.
Conclusion and future work
The development and regulatory approval of Transmission Raman methods for the routine QC testing will revolutionise how samples are analysed for assay, content uniformity and identification. These non-destructive Raman test methods require no sample preparation, standards, solvents, glassware, or consumable for routine QC analysis, making them very fast and simple alternatives to traditional liquid chromatography.
While routine use is straightforward and requires a very short training time, initial method development and calibration require suitable technical knowledge in order to build the chemometric model that relates the samples’ spectral features to the API amount, and to write the regulatory submission document.
Following these initial regulatory approvals for tablet products, review times for subsequent methods are likely to become shorter as regulatory acceptance becomes more widespread and the technique is applied to more sample types (Transmission Raman is not limited to tablets).
Actavis UK Ltd is actively developing further Transmission Raman methods for different solid dose products with the aim of reducing the laboratory costs and the time for release of products to the market.
References
- Validation of analytical procedures: text and methodology Q2(R1), 2005, International Conference on Harmonisation
- Guideline on the use of near infrared spectroscopy by the pharmaceutical industry and the data requirements for new submissions and variations, 2014, European Medicines Agency
- Guidelines of 16.05.2013 on the details of the various categories of variations, on the operation of the procedures laid down in Chapters II, IIa, III and IV of Commission Regulation (EC) No 1234/2008 of 24 November 2008 concerning the examination of variations to the terms of marketing authorisations for medicinal products for human use and veterinary medicinal products and on the documentation to be submitted pursuant to those procedures, European Commission.
Biographies
Julien Villaumié completed in PhD (2009) in Raman spectroscopy at Queen’s University Belfast, UK, under the supervision of Pr. Steven E.J. Bell. He is currently a R&D senior scientist at Actavis UK Ltd. Prior to joining Actavis in 2013, Julien worked in the pharmaceutical industry (Schering-Plough) and the medical devices industry (Abbott Vascular). His areas of expertise include quantitative analysis by Raman spectroscopy and Six Sigma.
Hilary Jeffreys graduated from the University of Aberdeen with a PhD in Chemistry. She has over 25 years of experience working in the technical field within the pharmaceutical industry. She is currently the Technical Transfer Manager at Actavis UK, leading teams responsible for the delivery of new products, technology and the development of technical skills including apprenticeships. She is taking a leading role in the development and promotion of skills in the scientific field and has won several national awards including Outstanding Leadership in Skills 2014 and Inspiration and Industry Award 2015.